
Effect of polymerization temperature on the mechanical properties of provisional prosthesis resins
Abstract
The purpose of this study was to examine the effects of the curing sequence and polymerization temperature on the flexural strength and microhardness of two provisional resins (Bis-acryl resin composite and polymethyl methacrylate (PMMA)). Polymerization was carried out under various conditions, in air at 25℃ (control) and in hot water (40, 50, 60, 70, and 80℃). The flexural strength test was conducted according to ISO-4049. The Knoop hardness was measured. For the Bis-acryl resin, the temperature up to 50℃ did not increase the flexural strength nor the hardness of the bis-acryl resin composite (p>0.05) but higher temperatures increased the strengths. For the PMMA resin, flexural strength increased with temperatures up to 70℃ and then decreased slightly. Bis-acryl resin composite had higher mechanical properties than the PMMA resin. The effect of heat was more pronounced in the bis-acryl resin composite than in the PMMA resin (p<0.05).
초록
본 연구의 목적은 2가지 임시 수지 (Bis-acryl resin composite 및 polymethyl methacrylate (PMMA))의 굴곡 강도 및 미세 경도에 대한 경화 순서 및 중합 온도의 영향을 조사하는 것에 있다. 중합은 대기 중 25℃ (대조) 및 온수 (40, 50, 60, 70 그리고 80℃)에서 다양한 조건하에 수행되었다. 굴곡 강도 시험은 ISO-4049에 따라 수행되었다. 또한, 누프 경도를 측정 하였다. Bis-acryl resin의 경우, 50℃까지의 온도는 굴곡 강도와 bis-acryl resin composite의 경도를 증가시키지 않았지만 (p> 0.05), 온도가 높을수록 강도가 증가 하였다. PMMA 수지의 경우 굴곡 강도는 최대 70℃의 온도에서 증가하고 약간 감소합니다. Bis-acryl resin은 PMMA 수지보다 기계적 특성이 더 컸다. 열의 영향은 Bis-acryl resin에서 PMMA 수지보다 더 두드러졌다(p <0.05).
Keywords:
Polymerization temperature, Mechanical properties, Bis-acryl resin, PMMA resin키워드:
중합 온도, 기계적 성질, 비스 아크릴 레진, 폴리메타메틸크릴레이트INTRODUCTION
Extensive prosthodontic treatment often requires fabrication of long-term provisional restorations. Fixed provisional restorations are also indicated for partially edentulous patients undergoing implant therapy when teeth adjacent to the edentulous area are restored with complete crowns (Galindo et al., 1998). For the purposes, provisional restorations must satisfy proper mechanical requirements to resist functional and nonfunctional loads (Karaokutan et al., 2015). However, breakage is still a potential problem of provisional resin restorations, especially when long term or long span provisional restorations are required. Breakage of the provisional restoration may lead to the loss of occlusion, periodontal health, and a change of the inter abutment relationship (Ogawa et al., 2000).
For the fabrication of single and multiple-unit provisional restorations, several types of resin-based materials have been employed. They include auto-polymerizing and dual curing resins, such as polymethyl methacrylate (PMMA), polyethyl methacrylate (PEMA), polyvinyl ethyl methacrylate (PVEMA), bis-GMA resins, bis-acryl resin composites, and visible light cured (VLC) urethane dimethacrylate resins (Burns et al., 2003; Karaokutan et al., 2015). They differ one another in terms of polymerization method, filler composition and monomer type. Historically, autopolymerizing PMMA resin has been the material most frequently used by clinicians for the direct and indirect fabrication of provisional fixed restorations (Christensen, 1996; Duke, 1999; Shillingburg et al., 1997; Burns et al 2003). Recently, bis-acryl resin composites have become a popular alternative choice for clinicians in the direct fabrication of provisional restorations (Duke, 1999).
However, the materials do not have sufficient mechanical properties to obtain long-term durability due to their poor strength (Karaokutan et al., 2015). Several techniques have been proposed to increase the strength and life of provisional restorations. For instance, provisional restorations can be reinforced with a metal framework (Galindo, 1998), stainless steel wire (Powell et al., 1994), or polyethylene or carbon graphite fibers (Larson et al 1991; Ramos et al., 1996). However, these studied focused not on the provisional materials, but on the external reinforcing.
Alternatively, the mechanical strength problems can be overcome by the heating of resin materials to a certain degree. Placing the provisional resin restorations in hot water is an accepted procedure and, often, is recommended in the manufacturers’ directions (Haselton, 2002). Therefore, the purpose of this study was to examine the effects of the curing sequence and polymerization temperature on the flexural strength and microhardness of provisional resins.
MATERIALS AND METHODS
Figure 1 gives the flow diagram of the process. Bis-acryl resin composite was dispensed via a cartridge system and allowed to autopolymerize, whereas methacrylate resin was handmixed and autopolymerized (Table 1). For each group, five bar-shaped specimens with dimensions of 25 × 2 × 2 mm were fabricated for flexural strength testing according to ISO 4049. Polymerization was carried out by a two-step method: 15 minutes in water followed by 15 min in air (WA) and 15 min in air followed by 15 min in water (AW). The hot water had temperatures varying between 40 and 80℃. In The control group, polymerization was done in air at 25℃ for 30 min (Table 2). After polymerization, the specimens were taken out of the molds and excess material was removed. The specimens were then stored in distilled water for 24 h at 37℃. Then the specimens were positioned on a testing apparatus with 20 mm support separation. Three-point bend test was performed using a universal testing machine (4200, Instron Inc., Canton, USA) with a 100 kg load cell and a crosshead speed of 1.5 mm/min. The flexural strength of each specimen (in MPa) was determined using the formula: S= 3WL/2bd2 , where W is the flexural load (Newton), L is the distance between supports (mm), b is the specimen width (mm) and d is the specimen thickness (mm) (Taylor & Lynch, 1992).
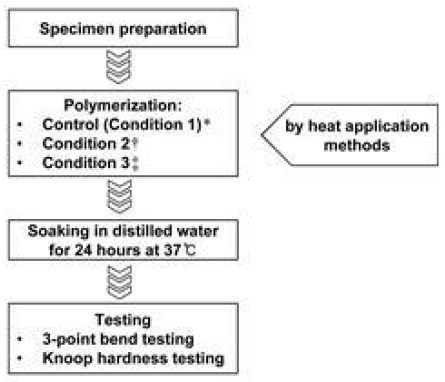
Flow chart of the sequence of operations involved in the present work.* : Condition 1: 30 min in air at 25℃ (control group).†: Condition 2: 15 min in Water followed by 15 min in Air (WA group).‡: Condition 3: 15 min in Air followed by 15 min in Water (AW group).
For the microhardness test, a mold (10 × 10 × 3 mm) was used to fabricate five specimens for each group. Polymerization was carried out as described above. After polymerization, the surface of the specimen was wet-polished using a 2000-grit abrasive paper and then tested. These values were obtained using microhardness tester (FM 7353, Future-tech Corp., Tokyo, Japan) using a 25 g load. Load was applied for a time of 10 s (five measurements for each specimen).
The means of flexural strengths and microhardness values were compared using one-way ANOVA with Tukey’s test at a significance level of 0.05. All statistical analysis was performed using SPSS 12.0 for Windows (SPSS Inc., USA).
RESULT
The results of flexural strength tests of the bis-acryl resin composite are given in Figure 2. No significant difference was found between 40 and 50℃ groups and the control. The flexural strength of WA60 was comparable to that of the control, whereas that of AW60 was higher than that of the control (p<0.05). At temperatures higher than 60℃, flexural strengths of both resins showed at least 30% increase compared to that of the control (p<0.05). Except for the 60℃ condition, no statistically significant differences in the value were found between the WA and AW groups for all the temperatures.
The results of flexural strength tests of the PMMA resin are given in Figure 3. Both the AW and WA groups had higher strengths than the control (p<0.05) for all the temperatures tested. The flexural strengths increased from the treatment temperature of 40℃ up to 70℃ and decreased at 80℃. Except for the 60℃ condition, the AW groups showed greater value than the WA groups (p<0.05).
The Knoop hardness of the bis-acryl resin composite polymerized under different conditions are shown in Figure 4. The hardness values of both the WA and AW group were the same under the treatment temperatures of 40 and 80℃. At the intermediate temperatures of 50, 60 and 70℃, the WA groups had higher hardness than the corresponding AW groups (p<0.05). The hardness of the AW groups was higher than the control only at the temperatures of 70℃ and above. The hardness of the WA groups was higher than the control even at 50℃.
Figure 5 gives the Knoop hardness of the PMMA resin. The hardness of the AW groups increased up to 50℃ and attains a plateau thereafter. The hardness of the WA groups was the same at 40 and 50℃, increased steeply at 60℃, was stable thereafter. Except for the 40℃ condition, the hardness of the WA groups was lower than the corresponding AW groups. The hardness of the AW groups was higher than the control at the temperatures of 50℃ and above. The hardness of the WA groups was higher than the control only from 60℃ onwards. The AW groups had higher hardness than the corresponding WA groups (p<0.05) at all temperatures, but except for the 40℃ conditon.
DISCUSSION
This study focused on the mechanical properties of polymerized PMMA resin and bis-acryl resin composite. The objective of this study wass to deduce the influence of polymerization sequence (air followed by hot water/water followed by air) on the mechanical properties. Differences in flexural strength and microhardness can be generally attributed to differences in chemical composition. Traditional MMA resins are monofunctional. They are low-molecular-weight, linear molecules that exhibit decreased strength and rigidity (Haselton et al., 2002). Base pastes of bis-acryl resin composite consist of bifunctional acrylates to provide cross-linkage for increased mechanical strength. In addition, the bis-acryl resin composite contain inorganic fillers to increase their resistance to abrasion and increase mechanical strength (Ireland et al., 1998).
In this study, bis-acryl resin composite showed flexural strength values in the range of 57.6-91.6 MPa for all the five temperatures tested. Compared to control, 40 and 50℃ treatment had lower flexural strengths, whereas 60℃ and higher temperature treatments increased the flexural strength.
As heat affects rate of the chemical reaction, temperature during polymerization may affect mechanical properties of resin. Resins polymerized at higher temperature may be stronger (Ogawa et al., 1999). No significant difference in strengths between the WA and AW groups was observed for all the treatment temperatures. Heat was given during initial stages of polymerization in the WA groups, whereas heat was given in the later stages of polymerization in the AW groups. Therefore, the effective degree of polymerization was same in both the cases, leading to similar flexural strengths. It was possible to produce various sequence of polymerization (WA or AW) in the laboratories. However, in clinical practice of making prosthesis for placement inside the mouth, it is not advisable to use the WA type polymerization because this method produces heat during the initial stages of polymerization. As the AW type also has similar flexural strengths, these procedures can be preferred.
Overall, the PMMA resin showed flexural strengths in the range of 59.6-72 MPa for all the five temperatures tested. Unlike the bis-acryl resin composite, even 40℃ treatment showed higher flexural strength than the control. In the case of bis-acryl resin composite, higher temperature treatment (80℃) increased the strength to 1.5 times that of control, whereas a similar treatment of the PMMA resin showed only 1.2 times that of the control. This indicates that the bis-acryl resin composite underwent a change to obtain even higher strengths. Of the two resins, bis-acryl resin composite may be a better choice in terms of flexural strength. Although our findings are similar to those of a previous study by Ogawa et al. (2015), the AW or WA samples were post-treated in water at 37℃ for 24 h and showed higher strengths, probably due to longer immersion times. The PMMA resin composition used in this study contains PMMA powder dispersed in the liquid. Higher the boiling point of the powder, more is the porosity and lower is the strength of the polymer. The powder boils at 100.8℃ (Usanmaz et al., 2003), whereas ethanol boils at 78℃. For the reason, the flexural strength at 80℃ was lower than that at 70℃. Except for the 60℃ condition, the PMMA-AW groups demonstrated greater flexural strengths than the corresponding WA groups. Initial polymerization leaves a thin hard polymer layer on the surface of the AW groups. This reduces the chance of volatilization or occurrence of voids in the polymer. Therefore, the flexural strengths of the AW group were high. However, in the case of WA group, no such hard layer was formed. Therefore, chances of ethanol volatilization resulted in more voids and pores, lowering the flexural strengths of the WA groups.
The PMMA resin exhibited significantly lower microhardness than the bis-acryl resin composite. The control bis-acryl resin composite had a hardness of 20 KHN and the polymers had a range of 19.1-24 KHN. In the case of PMMA resin, the control had a hardness of 12 KHN and the polymers had a range of 12.4-16.7 KHN. In the case of bis-acryl resin composite, the hardness of both AW and WA groups increased with increasing treatment temperatures. The WA groups had a lower hardness than the control group for up to 50℃. The WA groups produced higher hardness values than the AW groups, even at 50℃. This indicates that the sequence of polymerization is a key parameter to determine the mechanical properties. However, the AW groups had a lower hardness than the control for treatment temperatures as high as 70℃. During the WA polymerization, heat liberated during the initial stages of polymerization and formed a thin hard layer due to higher kinetics of the polymerization reaction. Such an effect is either absent or weak in the AW groups and the hardness increase is delayed. In the case of the PMMA resin, the hardness of both AW and WA types increased from 40 to 60℃ and then maintained. Unlike the bis-acryl, the PMMA-WA groups had a lower hardness than the AW groups. In addition, similar to the behavior observed in the case of the bis-acryl (Fig. 4), AW type PMMA polymerization produced higher hardness than the corresponding WA groups. This indicates that the sequence of polymerization influences greatly the mechanical properties. In the WA type of polymerization, though heat was liberated during initial stages, residual pores remained in the polymer. Therefore, the mobility was lowered and hardness was also low.
This study shows that increased heat (higher water temperatures) increased the flexural strength and microhardness. However, heat activation also increased shrinkage of the resin during polymerization. Provisional crowns polymerized in 20 or 30℃ water had much better margin adaptation than those fabricated in water at a higher temperature or those treated in air at 20℃ (Ogawa et al 1999). Therefore, a low water temperature is recommended. A sequence of operations like initial polymerization in water at 20 or 30℃ followed by polymerization in hot water (40℃ or higher) is expected to show a combination of high strength and hardness with low shrinkage.
CONCLUSION
Based on the findings of this study, the following conclusions are drawn:
1. The treatment temperature up to 50℃ did not increase the flexural strength nor the hardness of the bis-acryl resin composite. Higher treatment temperatures increased these values.
2. For the PMMA resin, water treatments increased flexural strength and hardness. Post hot water treatment gave more effect on the properties than the early hot water treatment.
3. The Bis-acryl resin composite had higher mechanical properties than PMMA resin. The effect of heat on these mechanical properties was more pronounced in the bis-acryl resin composite than in PMMA resin.
Acknowledgments
This research was supported by Basic Science Research Program through the National Research Foundation of Korea (NRF) funded by the Ministry of Education (NRF-2017R1A6A3A11036498).
References
-
Burns, DR, Beck, DA, Nelson, SK, (2003), A review of selected dental literature on contemporary provisional fixed prosthodontic treatment: report of the Committee on Research in Fixed Prosthodontics of the Academy of Fixed Prosthodontics, J Prosthet Dent, 90, p474-497.
[https://doi.org/10.1016/S0022-3913(03)00259-2]
-
Christensen, GJ, (1996), Provisional restorations for fixed prosthodontics, J Am Dent Assoc, 127, p249-251.
[https://doi.org/10.14219/jada.archive.1996.0177]
- Duke, ES, (1999), Provisional restorative materials: a technology update, Compend Contin Educ Den, 20, p497-500.
-
Galindo, D, Soltys, JL, Graser, GN, (1998), Long-term reinforced fixed provisional restorations, J Prosthet Dent, 79, p698-701.
[https://doi.org/10.1016/S0022-3913(98)70078-2]
-
Haselton, DR, (2002), Contemporary Fixed Prosthodontics, J Prosthodont, 11, p227-228.
[https://doi.org/10.1111/j.1532-849X.2002.223_4.x]
-
Haselton, DR, Diaz-Arnold, AM, Vargas, MA, (2002), Flexural strength of provisional crown and fixed partial denture resins, J Prosthet Dent, 87, p225-228.
[https://doi.org/10.1067/mpr.2002.121406]
-
Ireland, MF, Dixon, DL, Breeding, LC, Ramp, MH, (1998), In vitro mechanical property comparison of four resins used for fabrication of provisional fixed restorations, J Prosthet Dent, 80, p158-162.
[https://doi.org/10.1016/S0022-3913(98)70104-0]
-
Karaokutan, I, Sayin, G, Kara, O, (2015), In vitro study of fracture strength of provisional crown materials, J Adv Prosthodont, 7, p27-31.
[https://doi.org/10.4047/jap.2015.7.1.27]
-
Larson, WR, Dixon, DL, Aquilino, SA, Clancy, JM, (1991), The effect of carbon graphite fiber reinforcentent on the strength of provisional crown and fixed partial denture resins, J Prosthet Dent, 66, p816-820.
[https://doi.org/10.1016/0022-3913(91)90425-V]
-
Ogawa, T, Aizawa, S, Tanaka, M, Matsuya, S, Hasegawa, A, Koyano, K, (1999), Effect of water temperature on the fit of provisional crown margins during polymerization, J Prosthet Dent, 82, p658-661.
[https://doi.org/10.1016/S0022-3913(99)70006-5]
-
Ogawa, T, Tanaka, M, Koyano, K, of Dentistry, F, (2000), Effect of water temperature during polymerization on strength of autopolymerizing resin, J Prosthet Dent, 84, p222-224.
[https://doi.org/10.1067/mpr.2000.108574]
- Powell, DB, Nicholls, JI, Yuodelis, RA, Strygler, H, (1994), A comparison of wire-and Kevlar-reinforced provisional restorations, Int J Prosthodont, 7, p81-89.
-
Ramos, V, Runyan, DA, Christensen, LC, (1996), The effect of plasma-treated polyethylene fiber on the fracture strength of polymethyl methacrylate, J Prosthet Dent, 76, p94-96.
[https://doi.org/10.1016/S0022-3913(96)90348-0]
- Shillingburg, HT, Hobo, S, Whitsett, LD, Jacobi, R, Brackett, SE, (1997), Fundamentals of fixed prosthodontics, Book reviews J Prosthodont, 6, p316-320.
-
Taylor, MJ, Lynch, E, (1992), Microleakage, J Dent, 20, p3-10.
[https://doi.org/10.1016/0300-5712(92)90002-T]
-
Usanmaz, A, Ateş, J, Doğan, A, (2003), Thermal and mechanical properties of microwave‐and heat‐cured poly (methyl methacrylate) used as dental base material, J Appl Polym Sci, 90, p251-256.
[https://doi.org/10.1002/app.12681]