
Effects of RetroMTA on osteoblastic differentiation in MC3T3-E1 cells


Abstract
Mineral trioxide aggregate (MTA) provides a very good sealing, acceptable biocompatibility, dentin bridge formation and osteo-inductive effect. However, MTA has a few drawbacks. RetroMTA including calcium-zirconia complex has been developed to overcome drawbacks of MTA, especially long setting time and difficult handling characteristics. Therefore, the aim of this study was to evaluate the effects of RetroMTA on biocompatibility, osteogenic differentiation and mineralization in MC3T3-E1 cells compared with conventional MTA. Cytotoxicity was assessed by WST-1 assay. The gene expression of osteogenic markers was detected by real-time PCR. ALP activity and mineralization behavior were evaluated using ALP and alizarin red staining. There was no statistically significant difference between ProRoot MTA and RetroMTA with material extracts at dilutions of 1/4, 1/10 and 1/50 in cell viability assay. Expression of osteogenic markers in both ProRoot MTA and RetroMTA groups was significantly higher than in control group. In the mineralization assay, RetroMTA showed significantly higher formation of mineralized nodules than in control. In conclusion, RetroMTA promotes osteogenic differentiation and potential mineralization. It appears to be a potential alternative to ProRoot MTA as a root-end filling material.
초록
Mineral trioxide aggregate (MTA)는 좋은 밀폐성, 생체적합성, 상아질교 형성 및 골유도 효과 등의 여러 장점을 제공하지만 몇몇 단점들도 가지고 있다. 기존 MTA의 단점들, 특히 긴 경화시간과 어려운 조작성을 개선하기 위해서 칼슘-지르코니아 복합체를 함유한 RetroMTA가 개발되었다. 따라서 본 연구는 MC3T3-E1세포에서 생체적합성, 조골세포 분화능 및 광화에 대해 기존 MTA와 비교하여 RetroMTA의 효과를 평가하고자 하였다. WST-1 분석을 통해 세포독성평가를 시행하였고, 실시간 중합연쇄반응(real-time polymerase chain reaction)을 이용해 조골세포 분화 유전자를 확인하였다. 알칼리포스파타제 활성(Alkaline phosphatase activity) 및 광화에 대한 효과는 알칼리포스파타제 염색(Alkaline phosphatase staining) 및 알리자린 레드 염색(Alizarin red staining)으로 평가하였다. 세포독성평가에서 ProRoot MTA와 RetroMTA 추출물의 1/4, 1/10, 1/50 희석액에서 통계적으로 유의한 차이가 존재하지 않았다. ProRoot MTA와 RetroMTA군 모두에서 조골세포 분화인자들이 대조군에서보다 유의하게 높게 발현되었다. 광화분석실험에서는 RetroMTA가 대조군보다 유의하게 높은 무기물 결정 형성을 보였다. 결론적으로 RetroMTA는 조골세포 분화를 촉진시키고 광화를 유도하는 능력을 가지기 때문에 치근단 역충전재로서 ProRoot MTA의 잠재적인 대안이 될 수 있을 것이라 보인다.
Keywords:
MTA, RetroMTA, osteogenic differentiation, mineralization키워드:
조골세포분화, 광화INTRODUCTION
Mineral trioxide aggregate (MTA) is considered a “gold standard” for several endodontic applications such as pulp capping, repair of perforations or root resorption, apexification and apical surgery (De‐Deus et al. 2012). MTA provides a very good sealing (Barrieshi-Nusair and Hammad 2005), acceptable biocompatibility (Hwang et al. 2011), and dentin bridge formation in animals (Tziafas et al. 2002; Lee et al. 2017) or human teeth (Qudeimat et al. 2007). ProRoot MTA (Dentsply International Inc., Johnson City, TN, USA) is a conventional material, which is composed of tricalcium silicate, dicalcium silicate, tricalcium aluminate, calcium sulfate dehydrates and bismuth oxide (Shojaee et al. 2015), with a few drawbacks such as discoloration potential, presence of heavy metals (arsenic, chromium and lead), difficult handling characteristics, long setting time (Parirokh and Torabinejad 2010a; Parirokh and Torabinejad 2010b) and high material cost. The possibility of tooth discoloration associated with the use of MTA has been attributed to the presence of radiopacifying agents such as bismuth oxide, which raises a major clinical concern as it may negatively affect the patients’ anterior esthetics. Furthermore, MTA is exposed to blood. Because of the long setting time of the MTA, the MTA wash-out frequency increases in the blood. Unset MTA or washed-out MTA potentially results in unfavorable clinical outcomes (Kim et al. 2011).
Several new brands of MTA have been introduced into the market in an attempt to overcome these shortcomings. A new type of MTA (RetroMTA; BioMTA, Seoul, Korea) has recently been introduced in the market as a new hydraulic bioceramic material for similar endodontic applications as MTA. The manufacturer reported that elimination of Portland cement and its replacement with a new generation of nano-materials resulted in the elimination of toxic compounds and heavy metals from the conventional MTA. RetroMTA contains calcium zirconia complex as a radiopacifier. According to the manufacturer, RetroMTA is ideal for esthetic repair, since it has no discoloration and leads to rapid setting, which is beneficial in the moist environment of the oral cavity (Souza et al. 2015). Considering that the initial setting time of ProRoot MTA is about 165 min (Parirokh and Torabinejad 2010a), this is a substantially shorter time, and clinically effective because a retrograde filling material in apical surgery is expected to set quickly to avoid wash-out by blood or tissue fluid. In addition, since retrograde filling material is expected to be in permanent and close contact with periradicular tissues, it is important to assess its biocompatibility and biological effects in living cells (Zhou et al. 2013). Therefore, the aim of this study was to evaluate the cytotoxicity, effects of osteogenic differentiation and mineralization of RetroMTA compared with ProRoot MTA.
MATERIALS AND METHODS
1. Sample preparation and material extracts
Chemical compositions of ProRoot MTA and RetroMTA are presented in Table 1 (Kang et al. 2015). Both materials are mixed according to the manufacturer’s instructions.
The materials were placed into sterile cylindrical polyethylene tube (5 mm in diameter and 3 mm in height). To attain complete setting, the samples were incubated for 24 h at 37℃ and 95% relative humidity. After setting, both surfaces of samples were exposed to ultraviolet light (1 hour) for sterilization. Six specimens per test material immersed in 50 mL alpha-modification of Eagle’s Medium (α-MEM; Invitrogen, Carlsbad, CA, USA) were supplemented with 10% fetal bovine serum (FBS; Invitrogen) and 1% antibiotics (100 U/mL of penicillin and 100 μg/mL of streptomycin; Invitrogen) at 37℃ in a humidified atmosphere with 5% CO2 for 24 h. The extracts were collected and filtered using 0.20 μm filters (Minisart, Sartorius Stedim Biotech, Goettingen, Germany) for sterilization. The experimental group was treated with its material extract. The control group was treated with media only.
2. Cell culture
MC3T3-E1, a cell line derived from the calvaria of a C57BL/6 mouse embryo/fetus, was selected for this study and cultured in α-MEM supplemented with 10% FBS and 1% antibiotics in humidified atmosphere of 5% CO2 at 37℃.
3. Cell viability assay
The MC3T3-E1 cells were seeded into 96-well culture plates at a density of 1 × 104 cells per well and incubated in a growth medium (α-MEM supplemented with 10% FBS and 1% antibiotics) for 24 h for adhesion. The medium was replaced with 100 μL material extracts in the experimental groups and incubated for 24 h. The MC3T3-E1 cells and the growth medium were used as control. To compare the dose-response relationship, the material extracts were gradually diluted in the growth medium to five dilutions (1, 1/2, 1/4, 1/10 and 1/50). Ten microliters of the water-soluble tetrazolium salt-1 (WST-1) reagent (EZ-Cytox; Daeil Lab Service Co., Ltd., Seuol, Korea) was added to each well and incubated at 37℃ for 2 h. Optical densities were measured using multiwell spectrophotometer (VERSAmax multiplate reader; Molecular Devices, Sunnyvale, CA, USA) at a wavelength of 420 nm. The relative cell viability of the test material was calculated for each test material as a mean percentage of the control.
4. RNA isolation and quantitative real-time polymerase chain reaction (PCR)
The MC3T3-E1 cells were seeded in 6-well plates at a density of 2 x 105 cells per well and incubated in a growth medium for 24 h. The growth medium was replaced with a medium containing 1/4 dilution of material extracts. The unreacted cells (growth medium only) were used for control. After 1 to 3 days in culture, the total RNA was extracted using a TRIzol reagent (Invitrogen). The purity and quantity of the total RNA were determined using a spectrophotometer (Nanodrop 2000; Thermo Fisher Scientific, Waltham, MA, USA). Complementary DNA was synthesized using Maxime RT PreMix Kit (iNtRON Biotechnology, Seongnam, Korea). Each reaction consisted of an initial denaturation step at 95℃ for 1 min followed by three-step cycling: denaturation at 95℃ for 30 s, annealing at 55℃ for 30 s and extension at 72℃ for 30 s. After 25~30 cycles, a final extension was performed at 72℃ for 5 min. Primer sequences used for PCR are listed in Table 2.
Quantitative real-time PCR was performed by using the QuantiTect SYBR Green PCR kit (Qiagen, Valencia, CA, USA) on a 72-well Rotor-Gene 6000 (Corbett Research, Sydney, Australia). The mean cycle threshold values from triplicate measurements were used to determine the relative expression of the target gene with normalization to the endogenous control β-actin. The relative change in gene expression was analyzed by the 2-ΔΔCT (Livak and Schmittgen 2001).
5. ALP staining
The MC3T3-E1 cells were seeded in 24-well plates at a density of 2 × 104 cells per well with growth medium. After 24 h, the growth medium was changed to a medium containing 1/4 dilution of material extracts and cultured for 5 days. The cultured cells were washed with phosphate buffered saline (PBS) and fixed with 70% ethanol for 20 min at 4℃. After fixation, the cells were washed 3 times with deionized water and 300 μL ALP staining solution (NBT/BCIP Liquid Substrate System; Sigma-Aldrich, Saint Louis, MO, USA) was added to each well under dark conditions for 15 min. ALP staining was photographed and the staining density was quantified using an image analyzing program (Image J; National Institutes of Health, Bethesda, MD, USA).
6. Alizarin red staining
The mineralization effect was assessed by alizarin red staining. After the MC3T3-E1 cells were cultured with a growth medium containing 1/4 dilution of material extracts for 7 days, the cells were washed with PBS, and fixed with 70% ethanol for 1 h at 4℃. The cells were stained with 0.5% alizarin red (pH=4.2) for 10 min at room temperature with gentle agitation. The cells were rinsed with deionized water 5 times, rinsed with PBS for 15 min and air-dried. Alizarin red-stained cells were photographed and the staining density was quantified using an image analyzing program (Image J).
7. Statistical analysis
Each experiment, containing triplicate independent samples, was repeated at least three times, and qualitatively identical results were obtained. Student’s t test was used to assess differences in cell viability, ALP activity, and alizarin red staining. The results were considered significant at p < .05. For real-time PCR, 1-way analysis of variance (ANOVA) followed by Tukey post hoc test was used to determine statistically significant differences using SPSS 18.0 software program (SPSS, Chicago, IL, USA).
RESULTS
1. Cell viability
WST-1 assay was performed to determine the cell viability of MC3T3-E1 treated with different dilutions (1, 1/2, 1/4, 1/10 and 1/50) of material extracts. No statistically significant differences were observed between ProRoot MTA and RetroMTA when treated with 1/4, 1/10 and 1/50 dilutions of material extracts. None except the original material concentration was below the cut-off level (70%) established by ISO 10993-5 (ISO 2009) suggesting that all groups except the original concentration of the materials were biocompatible and improved cell viability compared with the positive control. Cell viability of the ProRoot MTA was significantly higher compared with RetroMTA at 1/2 and 1 dilutions of material extracts (p < .05) (Figure 1). Based on these data, the 1/4 dilution was used for the following experiments.
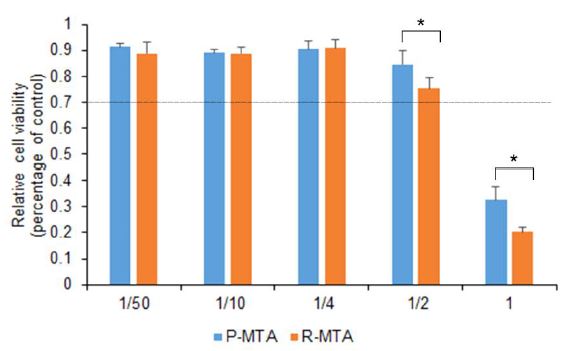
The cell viability of MC3T3-E1 cells exposed to extracts of the test materials. Cell viability of the ProRoot MTA was significantly higher compared with RetroMTA in the 1/2 and 1 dilutions of material extracts (*p < .05). Each value represents the mean ± SD of triplicate experiments. Dashed line represents the 70% cut-off level established by ISO 10993-5. (control: growth medium only; P-MTA: extracts of Proroot MTA, R-MTA: extracts of RetroMTA)
2. Quantitative real-time PCR
Real-time PCR was used to quantify the mRNA expression of ALP, BSP and OCN. No statistically significant differences were found between ProRoot MTA and RetroMTA for the mRNA expression of ALP, BSP and OCN on both days 1 and 3.
As shown in Figure 2A, the expression of ALP mRNA increased significantly in both ProRoot MTA and RetroMTA compared with the control (treated with medium only) on both days 1 and 3 (p < .05). However, no statistically significant differences existed between ProRoot MTA and RetroMTA on both days 1 and 3 (Figure 2A).
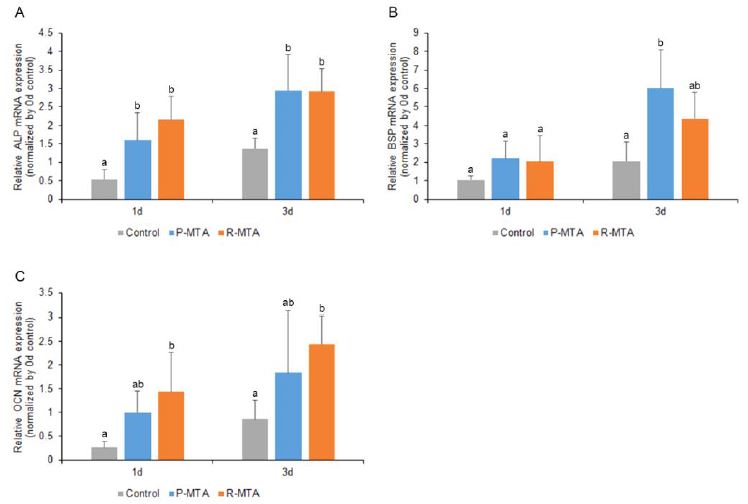
Effects of ProRoot MTA and RetroMTA on osteogenic differentiation in MC3T3-E1. Differentiation was determined by ALP (A), BSP (B) and OCN (C). Each value represents the mean ± SD of triplicate experiments. Different letters represent statistically significant differences between the test materials (p < .05); (control: growth medium only; P-MTA: extracts of Proroot MTA, R-MTA: extracts of RetroMTA).
In Figure 2B, the expression of BSP mRNA increased significantly in ProRoot MTA by day 3 compared with control but not RetroMTA (p < .05) (Figure 2B).
In Figure 2C, the expression of OCN mRNA increased significantly in RetroMTA compared with the control on days 1 and 3 (p < .05) (Figure 2C).
3. ALP activity and mineralization effect
We investigated ALP activity and mineralization effect of ProRoot MTA and RetroMTA following staining with ALP and alizarin red. According to the results of ALP staining (Figure 3A and C), the ProRoot MTA group showed significant increase in 5 days compared with the RetroMTA group (p < .05). Alizarin red staining showed that ProRoot MTA group increased mineralization significantly compared with the RetroMTA group at 7 days (p < .05).
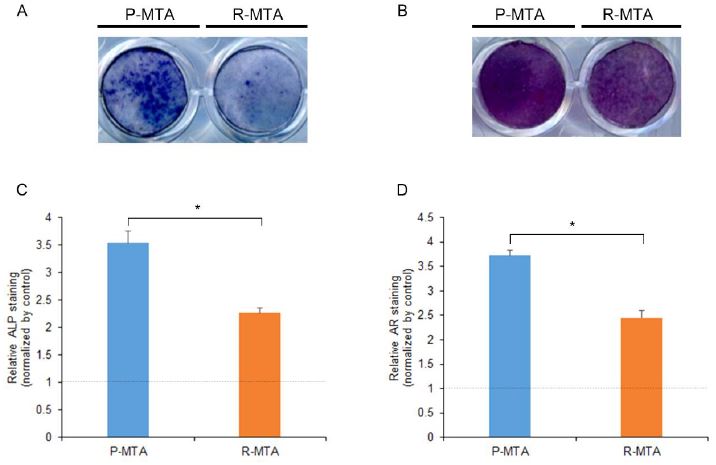
MC3T3-E1 cells stained with ALP and alizarin red following exposure to the extracts of the test materials. (A) ALP activity during osteogenic differentiation by the test materials in MC3T3-E1 cells on day 5. (B) Matrix mineralization of MC3T3-E1 cells observed by staining calcium deposits in the extracellular matrix on day 7. (C) Quantification of ALP staining data. (D) Quantification of alizarin red staining data. *p < .05, significant difference between ProRoot MTA and RetroMTA. Dashed line represents the control; (control: growth medium only; P-MTA: extracts of Proroot MTA, R-MTA: extracts of RetroMTA).
DISCUSSION
RetroMTA has been introduced commercially to overcome the limitations associated with conventional MTA. RetroMTA is characterized by a shorter initial setting time and better handling than ProRoot MTA and represented an effective substitute to ProRoot MTA. Therefore, we evaluated the biocompatibility and osteogenic effects of RetroMTA in this study.
In this study, cell viability was the lowest under the original concentration of ProRoot MTA and RetroMTA. Cell viability of the ProRoot MTA was significantly higher compared with RetroMTA at 1/2 and 1 dilutions of material extracts. These results were similar to those reported by other studies (Lee et al. 2015; Chung et al. 2016; Pornamazeh et al. 2017). The initial pH of RetroMTA was higher than that of the ProRoot MTA (Torabinejad et al. 1995b; Souza et al. 2015). MTA was cytotoxic when contacted directly (Torabinejad et al. 1995a; Camilleri et al. 2005; Koulaouzidou et al. 2008), which can be explained by the high pH during setting (Faraco and Holland 2001; Fridland and Rosado 2005). A high pH alters the integrity of the cytoplasmic membrane by disrupting organic components (proteins and phospholipids) and interfering with nutrient transport (Estrela et al. 1995). Therefore, cell viability might be reduced. Higher dilution of extracts resulted in a higher pH, which diminished the cell viability (Güven et al. 2013). However, the extracts from material are diluted with surrounding tissue fluids under clinical conditions. Therefore, the pH of the material extracts contacting cells may be actually lower clinically compared with in vitro studies, and had no effect on cell viability.
The differentiation of progenitor cells into osteoblast-like cells is critical in the healing process, and differentiation is required when considering a biomaterial as a root-end filling material. ALP is detected in the early differentiation phase and has an important function in mineral deposition (Wu et al. 2013). BSP is the major phosphorylated protein in a mammalian bone, with a possible role in the initiation of mineralization (Bianco et al. 1991; Hunter and Goldberg 1993). BSP is produced primarily by osteoblasts, and is considered a marker of the osteoblastic phenotype. BSP may serve as a matrix-associated signal that directly promotes osteoblast differentiation, resulting in increased production of mineralized matrix (Chen et al. 1992; Mizuno et al. 2000; Gordon et al. 2007). OCN is a major compound synthesized by osteoblasts, odontoblasts and cementoblasts (Nakashima 1994), and is a specific and relatively late-stage marker of osteoblastic differentiation (Malaval et al. 1994; Papagerakis et al. 2002). In this study, the expression of ALP, BSP and OCN mRNA in experimental groups was increased significantly compared with the control on both days 1 and 3. It is interesting that the expression of the early and late-stage specific markers in RetroMTA, especially, ALP and OCN mRNA in the RetroMTA group was higher than in the ProRoot MTA, without any statistically significant differences between RetroMTA and ProRoot MTA groups.
In the present study, based on ALP activity and alizarin red staining, additional mineralized nodules were generated in the ProRoot MTA group than in the RetroMTA group. Our results were similar to the results of a few previous studies (Chang et al. 2015; Lee et al. 2015). We performed the differentiation experiments using a growth medium. The induction medium was avoided because MTA showed excellent osteo-inductive effects (Lee et al. 2017; Yasukawa et al. 2017). We believe that these results could be explained by the pH of materials. MTA is capable of maintaining high pH over an extended period of time. The high pH induces the formation of a hard tissue. It has been suggested that the formation of mineralized tissue is also stimulated by the high pH of MTA (Torabinejad et al. 1995b; Okabe et al. 2006). Other studies support this suggestion by showing that the high alkalinity of the material plays a major role in the induction of a hard tissue barrier by creating a favorable environment for cell division, matrix formation and antimicrobial activity (Fridland and Rosado 2005; Maria de Lourdes et al. 2008). The pH value of ProRoot MTA is 10.2 after mixing, which increased to 12.5 at 1 h (Torabinejad et al. 1995b; Chng et al. 2005; Islam et al. 2006). ProRoot MTA maintained its high pH value (about 11~12) during the course of a long-term study. The authors attributed the high pH value to the constant release of calcium from MTA and the formation of calcium hydroxide (Fridland and Rosado 2005). However, according to the manufacturer, the pH of the RetroMTA is 12.5 after mixing, which decreased eventually to 7.8~8.0 in 4 weeks. We investigated ALP activity and alizarin red staining on days 5 and 7, respectively after mixing. Therefore, the pH value of the RetroMTA group might be lower than the pH value of the ProRoot MTA group. Accordingly, the degree of mineralization of ProRoot MTA group was higher than that of the RetroMTA group due to the effect of pH.
RetroMTA exhibited a short setting time due to the presence of zirconium, which accelerates the hydration of Portland cement (Li et al. 2013; Han et al. 2015). The short setting time of the RetroMTA also leads to a low wash-out frequency of the MTA, which was expected to be less affected by exposure to the blood vascular system. This observation was supported by studies in which the microhardness of RetroMTA was higher than that of ProRoot MTA when exposed to blood (Üstün et al. 2015; Song et al. 2016). The RetroMTA consists of increasingly fine and hydrophilic 2.62 μm particles than the ProRoot MTA (about 6.9 μm), suggesting that the RetroMTA has a superior handling property than ProRoot MTA (Parirokh and Torabinejad 2010a; Song et al. 2016). In addition, RetroMTA showed similar solubility compared with ProRoot MTA (Kwon et al. 2017). These features of the RetroMTA are a great advantage for apical surgery.
In this study, although the degree of mineralization of the RetroMTA was lower than that of the ProRoot MTA, RetroMTA might be a more appropriate choice in conditions in which blood contamination is unavoidable or in esthetically important areas of application. However, long-term evaluation via in vivo and clinical studies is needed.
CONCLUSION
RetroMTA showed good biocompatibility. The expression of osteogenic differentiation marker, ALP activity and mineralization potential were significant higher with RetroMTA compared with control. RetroMTA improves osteoblastic differentiation, and represents a desirable alternative to ProRoot MTA as a root-end filling material.
Acknowledgments
The authors deny any conflicts of interest related to this study.
Hyo-Il Lee and Sung-Hyeon Choi contributed equally to this work as first authors.
Bin-Na Lee and Won-Mann Oh contributed equally to this work as corresponding authors.
This study was supported by a grant (CRI 18029-1) of Chonnam National University Hospital Biomedical Research Institute and a grant (2016R1C1B1012703) of the National Research Foundation of Korea (NRF) grant funded by Ministry of Science, ICT and Future Planning (MSIP), Republic of Korea.
REFERENCES
- Barrieshi-Nusair, KM, Hammad, HM, (2005), Intracoronal sealing comparison of mineral trioxide aggregate and glass ionomer, Quintessence Int, 36, p539-545.
-
Bianco, P, Fisher, LW, Young, MF, Termine, JD, Robey, PG, (1991), Expression of bone sialoprotein (BSP) in developing human tissues, Calcif Tissue Int, 49, p421-426.
[https://doi.org/10.1007/bf02555854]
-
Camilleri, J, Montesin, FE, Di Silvio, L, Pitt Ford, TR, (2005), The chemical constitution and biocompatibility of accelerated Portland cement for endodontic use, Int Endod J, 38, p834-842.
[https://doi.org/10.1111/j.1365-2591.2005.01028.x]
-
Chang, SW, Bae, WJ, Yi, JK, Lee, S, Lee, DW, Kum, KY, Kim, EC, (2015), Odontoblastic differentiation, inflammatory response, and angiogenic potential of 4 calcium silicate–based cements: Micromega MTA, ProRoot MTA, RetroMTA, and experimental calcium silicate cement, J Endod, 41, p1524-1529.
[https://doi.org/10.1016/j.joen.2015.04.018]
- Chen, J, Shapiro, HS, Sodek, J, (1992), Developmental expression of bone sialoprotein mRNA in rat mineralized connective tissues, J Bone Miner Res, 7, p987-997.
-
Chng, HK, Islam, I, Yap, AU, Tong, YW, Koh, ET, (2005), Properties of a new root-end filling material, J Endod, 31, p665-668.
[https://doi.org/10.1097/01.don.0000157993.89164.be]
-
Chung, CJ, Kim, E, Song, M, Park, JW, Shin, SJ, (2016), Effects of two fast-setting calcium-silicate cements on cell viability and angiogenic factor release in human pulp-derived cells, Odontology, 104, p143-151.
[https://doi.org/10.1007/s10266-015-0194-5]
- De‐Deus, G, Canabarro, A, Alves, GG, Marins, JR, Linhares, AB, Granjeiro, JM, (2012), Cytocompatibility of the ready‐to‐use bioceramic putty repair cement iRoot BP Plus with primary human osteoblasts, Int Endod J, 45, p508-513.
- Estrela, C, Sydney, GB, Bammann, LL, Felippe Jr, O, (1995), Mechanism of action of calcium and hydroxyl ions of calcium hydroxide on tissue and bacteria, Braz Dent J, 6, p85-90.
-
Faraco, IM, Holland, R, (2001), Response of the pulp of dogs to capping with mineral trioxide aggregate or a calcium hydroxide cement, Dent Traumatol, 17, p163-166.
[https://doi.org/10.1034/j.1600-9657.2001.170405.x]
-
Fridland, M, Rosado, R, (2005), MTA solubility: a long term study, J Endod, 31, p376-379.
[https://doi.org/10.1097/01.don.0000140566.97319.3e]
- Güven, EP, Taşlı, PN, Yalvac, ME, Sofiev, N, Kayahan, MB, Sahin, F, (2013), In vitro comparison of induction capacity and biomineralization ability of mineral trioxide aggregate and a bioceramic root canal sealer, Int Endod J, 46, p1173-1182.
-
Gordon, JA, Tye, CE, Sampaio, AV, Underhill, TM, Hunter, GK, Goldberg, HA, (2007), Bone sialoprotein expression enhances osteoblast differentiation and matrix mineralization in vitro, Bone, 41, p462-473.
[https://doi.org/10.1016/j.bone.2007.04.191]
-
Han, L, Kodama, S, Okiji, T, (2015), Evaluation of calcium-releasing and apatite‐forming abilities of fast‐setting calcium silicate‐based endodontic materials, Int Endod J, 48, p124-130.
[https://doi.org/10.1111/iej.12290]
-
Hunter, GK, Goldberg, HA, (1993), Nucleation of hydroxyapatite by bone sialoprotein, Proc Natl Acad Sci U S A, 90, p8562-8565.
[https://doi.org/10.1073/pnas.90.18.8562]
-
Hwang, YC, Kim, DH, Hwang, IN, Song, SJ, Park, YJ, Koh, JT, Son, HH, Oh, WM, (2011), Chemical constitution, physical properties, and biocompatibility of experimentally manufactured Portland cement, J Endod, 37, p58-62.
[https://doi.org/10.1016/j.joen.2010.09.004]
-
Islam, I, Chng, HK, Yap, AU, (2006), Comparison of the physical and mechanical properties of MTA and Portland cement, J Endod, 32, p193-197.
[https://doi.org/10.1016/j.joen.2005.10.043]
- ISO, E, (2009), Biological Evaluation of Medical Devices. Part 5: Tests for In Vitro Cytotoxicity, International Organization for Standardization: Geneva, Switzerland, p10993-10995.
-
Kang, CM, Kim, SH, Shin, Y, Lee, HS, Lee, JH, Kim, GT, Song, JS, (2015), A randomized controlled trial of ProRoot MTA, OrthoMTA and RetroMTA for pulpotomy in primary molars, Oral Dis, 21, p785-791.
[https://doi.org/10.1111/odi.12348]
-
Kim, YR, Lee, CY, Kim, ES, Jung, IY, (2011), Failure of orthograde MTA filling: MTA wash-out?, Restor Dent Endod, 36, p510-514.
[https://doi.org/10.5395/jkacd.2011.36.6.510]
-
Koulaouzidou, EA, Economides, N, Beltes, P, Geromichalos, G, Papazisis, K, (2008), In vitro evaluation of the cytotoxicity of ProRoot MTA and MTA Angelus, J Oral Sci, 50, p397-402.
[https://doi.org/10.2334/josnusd.50.397]
-
Kwon, YD, Seok, S, Lee, SH, Lim, BS, (2017), Comparison of physical properties between paste type mineral trioxide aggregates (MTA and powder-liquid mix type MTA, Korean J Dent Mater, 44, p11-20.
[https://doi.org/10.14815/kjdm.2017.44.1.011]
-
Lee, BN, Song, YS, Lee, GW, Kim, YH, Chang, HS, Hwang, YC, Oh, WM, Hwang, IN, (2017), Comparison of TheraCal LC, mineral trioxide aggregate, and formocresol as pulpotomy agents in rat molar, Korean J Dent Mater, 44, p187-195.
[https://doi.org/10.14815/kjdm.2017.44.2.187]
-
Lee, BN, Chun, SJ, Chang, HS, Hwang, YC, Hwang, IN, Oh, WM, (2017), Physical properties and biological effects of mineral trioxide aggregate mixed with methylcellulose and calcium chloride, J Appl Oral Sci, 25, p680-688.
[https://doi.org/10.1590/1678-7757-2017-0050]
-
Lee, H, Shin, Y, Jung, J, Kim, S, Lee, J, Song, J, (2015), Biologic response of human deciduous dental pulp cells on newly developed MTA-like materials, J Korean Acad Pediatr Dent, 42, p291-301.
[https://doi.org/10.5933/jkapd.2015.42.4.291]
-
Li, Q, Deacon, AD, Coleman, NJ, (2013), The impact of zirconium oxide nanoparticles on the hydration chemistry and biocompatibility of white Portland cement, Dent Mater J, 32, p808-815.
[https://doi.org/10.4012/dmj.2013-113]
-
Livak, KJ, Schmittgen, TD, (2001), Analysis of relative gene expression data using real-time quantitative PCR and the 2−ΔΔCT method, Methods, 25, p402-408.
[https://doi.org/10.1006/meth.2001.1262]
-
Malaval, L, Modrowski, D, Gupta, AK, Aubin, JE, (1994), Cellular expression of bone‐related proteins during in vitro osteogenesis in rat bone marrow stromal cell cultures, J Cell Physiol, 158, p555-572.
[https://doi.org/10.1002/jcp.1041580322]
- Accorinte Mde, L, Holland, R, Reis, A, Bortoluzzi, MC, Murata, SS, Dezan, E Jr, Souza, V, Alessandro, LD, (2008), Evaluation of mineral trioxide aggregate and calcium hydroxide cement as pulp-capping agents in human teeth, J Endod, 34, p1-6.
-
Mizuno, M, Imai, T, Fujisawa, R, Tani, H, Kuboki, Y, (2000), Bone sialoprotein (BSP) is a crucial factor for the expression of osteoblastic phenotypes of bone marrow cells cultured on type I collagen matrix, Calcif Tissue Int, 66, p388-396.
[https://doi.org/10.1007/s002230010078]
-
Nakashima, M, (1994), Induction of dentin formation on canine amputated pulp by recombinant human bone morphogenetic proteins (BMP)-2 and-4, J Dent Res, 73, p1515-1522.
[https://doi.org/10.1177/00220345940730090601]
-
Okabe, T, Sakamoto, M, Takeuchi, H, Matsushima, K, (2006), Effects of pH on mineralization ability of human dental pulp cells, J Endod, 32, p198-201.
[https://doi.org/10.1016/j.joen.2005.10.041]
-
Papagerakis, P, Berdal, A, Mesbah, M, Peuchmaur, M, Malaval, L, Nydegger, J, Simmer, J, Macdougall, M, (2002), Investigation of osteocalcin, osteonectin, and dentin sialophosphoprotein in developing human teeth, Bone, 30, p377-385.
[https://doi.org/10.1016/s8756-3282(01)00683-4]
- Parirokh, M, Torabinejad, M, (2010a), Mineral trioxide aggregate: a comprehensive literature review—part I: chemical, physical, and antibacterial properties, J Endod, 36, p16-27.
- Parirokh, M, Torabinejad, M, (2010b), Mineral trioxide aggregate: a comprehensive literature review—part III: clinical applications, drawbacks, and mechanism of action, J Endod, 36, p400-413.
- Pornamazeh, T, Yadegari, Z, Ghasemi, A, Sheykh-Al-Eslamian, SM, Shojaeian, S, (2017), In Vitro Cytotoxicity and Setting Time Assessment of Calcium-Enriched Mixture Cement, Retro Mineral Trioxide Aggregate and Mineral Trioxide Aggregate, Iran Endod J, 12, p488-492.
-
Qudeimat, MA, Barrieshi-Nusair, KM, Owais, AI, (2007), Calcium hydroxide vs mineral trioxide aggregates for partial pulpotomy of permanent molars with deep caries, Eur Arch Paediatr Dent, 8, p99-104.
[https://doi.org/10.1007/bf03262577]
- Shojaee, NS, Sahebi, S, Karami, E, Sobhnamayan, F, (2015), Solubility of two root-end filling materials over different time periods in synthetic tissue fluid: a comparative study, J Dent, 16, p189-194.
-
Song, M, Yue, W, Kim, S, Kim, W, Kim, Y, Kim, JW, Kim, E, (2016), The effect of human blood on the setting and surface micro-hardness of calcium silicate cements, Clin Oral Investig, 20, p1997-2005.
[https://doi.org/10.1007/s00784-015-1693-z]
-
Souza, LC, Yadlapati, M, Dorn, SO, Silva, R, Letra, A, (2015), Analysis of radiopacity, pH and cytotoxicity of a new bioceramic material, J Appl Oral Sci, 23, p383-389.
[https://doi.org/10.1590/1678-775720150065]
- Torabinejad, M, Hong, CU, Pitt Ford, TR, Kettering, JD, (1995a), Cytotoxicity of four root end filling materials, J Endod, 21, p489-492.
- Torabinejad, M, Hong, CU, McDonald, F, Pitt Ford, TR, (1995b), Physical and chemical properties of a new root-end filling material, J Endod, 21, p349-353.
-
Tziafas, D, Pantelidou, O, Alvanou, A, Belibasakis, G, Papadimitriou, S, (2002), The dentinogenic effect of mineral trioxide aggregate (MTA) in short‐term capping experiments, Int Endod J, 35, p245-254.
[https://doi.org/10.1046/j.1365-2591.2002.00471.x]
- Üstün, Y, Topçuoğlu, HS, Akpek, F, Aslan, T, (2015), The effect of blood contamination on dislocation resistance of different endodontic reparative materials, J Oral Sci, 57, p185-190.
-
Wu, J, Jia, Q, He, W, Liu, J, Hou, L, Zhang, J, Niu, Z, Ni, L, (2013), Conditioned medium from periapical follicle cells induces the odontogenic differentiation of stem cells from the apical papilla in vitro, J Endod, 39, p1015-1022.
[https://doi.org/10.1016/j.joen.2013.04.011]
- Yasukawa, T, Hayashi, M, Tanabe, N, Tsuda, H, Suzuki, Y, Kawato, T, Suzuki, N, Maeno, M, Ogiso, B, Involvement of the calcium-sensing receptor in mineral trioxide aggregate-induced osteogenic gene expression in murine MC3T3-E1 cells, Dent Mater J, 36, p469-475.
-
Zhou, HM, Shen, Y, Wang, ZJ, Li, L, Zheng, YF, Häkkinen, L, Haapasalo, M, (2013), In vitro cytotoxicity evaluation of a novel root repair material, J Endod, 39, p478-483.
[https://doi.org/10.1016/j.joen.2012.11.026]