
표면조도가 치과수복용 CAD/CAM 세라믹-고분자 블록의 기계적 물성에 미치는 영향
초록
본 연구에서는 세라믹-고분자 CAD/CAM 블록의 표면 조도가 기계적 물성에 미치는 영향을 관찰하였다. 상용제품인 Polyglass와 Enamic을 사용하여 절삭 가공 후 6 μm와 1 μm의 다이아몬드 페이스트로 연마하여 3가지 표면조도를 갖는 시편을 제조하였다. 통계적 유의성은 유의수준 0.05에서 분산분석 및 대응표본 t-검정하였다.
6 μm 페이스트로 연마하면 평균 거칠기는 47~49%, 최대 거칠기는 68~69%로 감소하고, 1 μm 페이스트로 연마하면 평균 거칠기가 13~22%, 최대 거칠기는 16~19%로 더욱 감소하였다.
Polyglass와 Enamic 모두 표면조도가 감소함에 따라 굴곡강도, 와이블 계수, 탄성율, 파괴인성이 증가하였다. Polyglass의 기계적 물성은 P3까지 계속 증가하였으나, Enamic은 E2와 E3 간에 유의차가 없었다.
따라서 고분자 매트릭스 구조에 세라믹 입자가 분산강화된 Polyglass는 표면조도가 낮을수록 기계적 물성이 증가하지만, 세라믹 매트릭스에 고분자가 함침된 Enamic은 6 μm의 다이아몬드 페이스트로 연마하면 기계적 물성의 최대값을 나타내기에 충분하였음을 알 수 있었으며, 명확한 결론을 위해서는 계속적인 연구가 필요하다.
Abstract
The effect of surface roughness of ceramic-polymer CAD/CAM blocks on the mechanical properties was investigated in this study. Commercially available Polyglass (Vericom, Korea) and Enamic (Vita, Germany) were selected for this purpose. They were cut into either (4.0×2.1×17.0) mm and (3.0×4.0×17.0) mm, followed by grinding, and polished sequentially with 6 μm and 1 μm diamond paste. Flexural strength, fracture toughness, and Weibull analysis were determined according to ISO 6872 Dentistry-Ceramic materials. The elastic moduli were calculated from a stress-strain curves under flexural loading. The statistical significances of the mechanical properties between the products and surface roughness were analyzed with ANOVA and pared t-test at a significance level of 0.05. After grinding with 6 μm diamond paste after cutting by observing with an atomic force microscope, the arithmetic average roughness decreased to 47~49% and the maximum roughness decreased to 68~69%. When polishing with 1 μm diamond paste, The average roughness decreased to 13~22% and the maximum roughness decreased to 16~19%. When the flexural load was applied, stress increased linearly and fractured without plastic deformation both Polyglass and Enamic. As the surface roughness decreased, the mechanical properties were increased both Polyglass and Enamic. However, the mechanical properties of Polyglass increased up to P3, while Enamic showed almost maximal values at E2, after that there was no significant differences between E2 and E3. It could be due to the different microstructure between two blocks used in this experiment.
Keywords:
CAD/CAM Block, Surface roughness, Strength, Weibull analysis, Fracture toughness키워드:
CAD/CAM 블록, 표면조도, 강도, 와이블 분석, 파괴인성서 론
CAD/CAM 기술은 1977년 Young과 Altschuler에 의해 최초로 치과에 도입되었다. 광학 계측기를 이용하여 구강내 그리드 표면 지도 시스템(intraoral grid surface mapping system)을 개발한 것이다(Liu, 2005). 이후 Mormann과 Brandestini가 CEREC (Chairside Economical Restorations of Esthetic Ceramics) 시스템을 개발하여 치과에서 본격적으로 사용되기 시작하였다(Miyazaki 등, 2009).
CAD/CAM 기술로 치과 수복물을 만들기 위해서는 스캐닝부터 가공까지 전 공정에서 세밀한 제어가 필요하며, 현재 CEREC 시스템은 4세대까지 발전하고 있으며, Procera, DCS Precident, Cercon, Lava, ZenoTec, Everest, CeraSys, Wol-Ceram, DenZir, DentaCAD, etkon, TurboDent, Kataba 등의 다양한 CAD/CAM 시스템이 세계적으로 널리 사용되고 있다(Liu, 2005).
CAD/CAM은 제작소요 기간이 짧아서 환자의 만족도가 높고, 초기 투자비용을 제외하면 제작비용이 경제적이며, 일관된 특성을 가지는 제품을 무한정으로 제작할 수 있다. 또한 예전의 기공 작업으로는 사용이 거의 불가능했던 재료들을 CAD/CAM 기술로는 쉽게 사용할 수 있게 되었다. 재래식 기공 작업으로 14시간이 소요되던 InCeram 수복물을 CAD/CAM 기술로는 20분 내에 밀링하고, 40분~4시간에 유리 침투과정으로 제작할 수 있는 것이 대표적인 예이다. 이렇게 세라믹 재료를 쉽게 사용할 수 있게 되면서 향상된 물성을 갖는 치과 수복물이 가능해졌다 (Miyazaki 등, 2009). 또한, CAD/CAM으로 제작한 수복물은 파일이 저장되어 있어서 필요한 경우동일한 수복물을 쉽게 다시 만들 수 있다는 장점도 있다.
이상적 치과 수복을 위해서는 손상된 치아를 구조 및 물성이 유사한 재료로 대체하는 것이 유리하며, 특히 심미성에 대한 관심이 높아지면서 생체친화성과 화학적 내구성이 우수할 뿐 아니라, 자연치아와 색조가 유사한 세라믹 수복물의 수요가 크게 증가하고 있다(Albero 등, 2015; Miyazaki 등, 2009; Nguyen 등, 2012; Ruse와 Sadoun, 2014; Zahran 등, 2008). 하지만 세라믹은 취성(brittleness)의 고질적 한계로 가공공정 중에 치핑(chipping) 될 수 있으며, 임상 사용 중에도 예측하기 어려운 파절 가능성이 있을 수 있다.
세라믹의 우수한 심미성 및 강도를 고분자의 용이한 가공성 및 우수한 파괴인성과 복합하여 세라믹의 한계를 극복하고자 개발된 것이 세라믹-고분자 복합 블록이다(Spitznagel 등, 2014; Coldea 등, 2013; He와 Swain, 2011; Nguyen 등, 2014). 세라믹 CAD/CAM 블록은 한 세트의 버 (bur)로 최대 10개 정도만 가공할 수 있는데 비해, 세라믹-고분자 CAD/CAM 블록은 100개 이상 가공할 정도로 가공성이 뛰어나며, 치핑도 훨씬 적다고 한다(He와 Swain, 2011; Tsitrou 등, 2007).
치과 수복물의 교합 조정이 필요할 때 핸드피스로 표면을 연마(polishing)하면 글레이징(glazing) 표면보다 거칠어지고, 리글레이징(reglazing)하면 연마한 것보다는 표면 조도(surface roughness)를 훨씬 감소시킬 수 있다고 알려져 있으나 (Brewer 등, 1990; Chu 등, 2000; Fuzzi 등, 1996), 연마만으로도 글레이징한 것과 유사하거나, 더 매끄럽게 표면을 처리할 수도 있다는 연구 보고도 있다(Nakamura 등, 2010; Tholt 등, 2006; Wright 등, 2004).
표면에 결함(flaw)이 있는 세라믹 수복물을 임상에 적용하면 구강 내 수분에 의해 느린 균열 성장이 진행되어 갑자기 파괴될 수 있다(Rodrigues 등, 2008). 이와 같은 기전으로 수복물에 과도한 교합력이 가해지지 않는 경우에도 정적 피로에 의해 파괴가 일어날 수 있기 때문에(Lohbauer 등, 2008; White, 1993), 교합 조정 후 수복물의 연마는 수복물의 임상 수명에 매우 중요한 요소이다(Bollen 등, 1997). 컴포짓레진 수복물은 시간이 경과함에 따라 구강 내 수분이 필러와 고분자 계면를 통해 침투하여 확산됨에 따라 고분자 기질이 가수 분해되어 조성 성분이 용출되고 고분자가 가소화(plasticizing)되면서 물성이 저하될 있다고 한다(Ferracane, 2006). 이러한 과정은 pH 변화(Bagheri 등, 2007), 타액 효소(Jaffer 등, 2002) 및 습도(Ferracane, 1994; Groger 등, 2006; Ortengren 등, 2001) 등 구강 내 조건에 영향을 받는다.
세라믹-고분자 CAD/CAM 블록은 Paradigm MZ100 (3M ESPE, 미국)이 최초 출시된 제품이며, 이와 유사한 제품으로 Polyglass(베리콤, 한국)가 있다. 또 다른 제품은 고분자 침투 세라믹 망상(polymer-infiltrated-ceramic network) 구조를 갖는 Enamic (Vita, 독일)이 있는데, 이와 유사제품은 현재 개발 중으로 아직 상품으로 출시된 것은 없다. 이러한 두 가지 제품은 미세구조가 달라서 서로 다른 기계적 물성을 갖는다는 것이 최근 보고되었다(박종환, 2017).
본 연구에서는 고분자 매트릭스에 세라믹 필러가 분산된 구조를 갖는 CAD/CAM 제품과 세라믹 매트릭스에 고분자를 침투시킨 구조를 갖는 CAD/CAM 제품의 표면 조도를 변화시키면서 굴곡강도, 와이블 특성강도 및 계수, 탄성율, 파괴 인성 등의 기계적 물성에 미치는 영향을 규명하고자 하였다.
재료 및 방법
1. 실험재료
상용 CAD/CAM 블록인 Polyglass (Vericom Co., Korea)와 Enamic (Vita, Germany)의 두 가지 제품을 사용하였다. CNC machine (Samsung Machine Tools. Korea)을 이용하여 (4.0×2.1×17.0) mm 및 (3.0×4.0×17.0) mm의 두가지 크기로 절삭(cutting)한 후에, grinding machine (HRG 150, AM technology, Korea)으로 가공(grinding)하고, lapping machine (SPL-15, Grind-X, Okamoto, Japan)을 이용하여 6 μm와 1 μm의 다이아몬드 페이스트로 순차적으로 연마(polishing)하여 3가지 표면조도를 갖는 시편을 제조하였다. 제품 및 시편 준비 방법에 따라 실험군은 Table 1에 나타낸 것과 같이 분류하였다.
2. 실험방법
Atomic Force Microscope (XE-100, Park Systems, Korea)을 이용하여 가공과 연마에 따른 세라믹-고분자 블록의 최대 거칠기 (Rz)와 산술평균 거칠기 (Ra)를 측정하였다.
굴곡강도는 ISO 6872:2015 Dentistry - Ceramic materials에 따라 만능시험기(3366, Instron, USA)을 이용하여 span 12 mm의 지지대 중앙에 시편을 올려놓고, 1.0 mm/min의 속도로 하중을 가하여 최대 하중을 구한 후 아래 식 (1)로 3점 굴곡강도를 계산하였다.
(1) |
여기서, σ는 굴곡강도(MPa), P는 최대 하중(N), L은 span 길이(mm), b와 h는 각각 시편의 폭과 두께(mm)이다.
2.3 와이블 분석
굴곡강도의 와이블 분석은 ISO 6872의 Annex B. Weibull statistics에 따라 시행하였다. 굴곡강도 데이타를 오름차순으로 정리하여 순위를 매긴 후에 아래 식 (2)에 따라 파괴확률을 계산하였다.
(2) |
여기서, Pf는 파괴확률이고, i는 오름차순으로 정리한 순위, N은 시편의 개수이다.
아래 식 (3)으로부터 와이블 계수(modulus) m과 특성강도를 구하였다.
(3) |
2.4 탄성율 측정
탄성율은 ISO 13061-4:2014 Physical and mechanical properties of wood - Test methods for small clear wood specimens - Part 4: Determination of modulus of elasticity in static bending에 따라 굴곡시험으로 얻은 응력-변형율 곡선으로부터 아래 식 (4)로 계산하였다.
(4) |
여기서, E는 탄성율(MPa), P는 최대 하중 (N), L은 span 길이(mm), b와 h는 각각 시편의 폭과 두께(mm), f는 최대 변형량(mm)이다.
2.5 파괴인성 측정
파괴인성은 ISO 6872의 Annex A. Fracture toughness에 따라 단일 에지 V노치 빔(single-edge V-notch beam) 방법으로 측정하였다(ISO 23146). 다이아몬드 휠(wheel)과 페이스트를 이용하여 깊이가 0.8~1.2 mm이고, 곡률반경이 30° 이하인 노치를 만든 후, 노치가 아래쪽을 향하도록 만능 시험기(3366, Instron, USA)의 지지대 위에 올려놓고, 0.5 mm/min의 속도로 하중을 가하여 최대 하중을 구한 후 아래 식 (5)로 파괴인성을 계산하였다(Figure 1).
(5) |
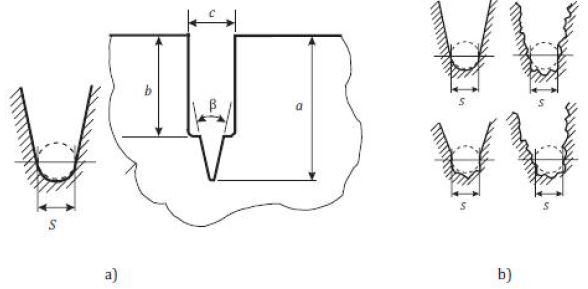
Schematic geometry of V-notch preparation: (a) notch width and angle measurement (b) interpretation of notch root shape according to ISO 23146.Keya 0.8 up to 1.2 mmb approximately 0.5 mmc width of razor blade, a-b > cβ approximately 30 degree (°) or as small as possibleS V-notch width
여기서, KIC는 파괴인성(MPa√m), F는 최대 하중(MN), S는 span 길이(m), α는 노치의 상대 깊이, Y는 응력확대형 상계수, b와 w는 각각 시편의 폭과 두께(m)이다.
노치의 상대 깊이는 3회 측정한 깊이로부터 아래 식 (6)과 같이 계산하였다.
(6) |
응력확대형상계수는 아래 식 (7)과 같이 계산하였다.
(7) |
2.6 통계 분석
제품 간의 기계적 물성의 통계적 유의성은 유의수준 0.05에서 분산분석(ANOVA) 및 대응표본 t-검정(paired t-test)하였다.
연구 결과
Atomic Force Microscope으로 관찰한 표면은 Figure 2와 같으며, 이로부터 측정한 최대 거칠기(Rz)와 산술평균 거칠기(Ra)를 Table 2에 나타내었다. 가공 후 6 μm의 다이아몬드 페이스트로 연마하면 산술평균 거칠기는 47~49%로 감소하고, 최대 거칠기는 68~69%로 감소하였다가, 1 μm의 다이아몬드 페이스트로 연마 후에는 산술평균 거칠기가 13~22%로 감소하고, 최대 거칠기는 16~19%로 감소하였다.
굴곡 하중 하에서의 응력-변형율 곡선을 Figure 3에 나타내었다. 응력이 증가함에 따라 Polyglass와 Enamic 모두 표면조도에 상관없이 변형이 직선적으로 증가하다가 파괴되는 양상을 나타내었다.
Figure 3의 응력-변형율 곡선으로부터 파괴될 때의 최대 응력으로부터 굴곡강도를 계산하여 Table 3에 나타내었다. 모든 표면조도에서 Polyglass가 Enamic보다 높은 굴곡강도를 나타내었으며, 1군과 3군에서는 유의한 차이가 있었다(p<0.05). Polyglass는 표면조도가 감소함에 따라 굴곡강도가 증가하여 P3에서 136.3±7.2 MPa의 최대 굴곡강도를 나타내었으며, 이는 P1 및 P2보다 유의하게 높았다(p<0.05). Enamic은 E2는 E1보다 유의하게 높은 117.7±7.4 MPa의 굴곡강도를 나타내었으나 (p<0.05), E3는 E2와 유사한 117.2±11.2 MPa를 나타내었다.
또한, Table 3에 명시된 탄성율 결과를 바탕으로 모든 표면조도에서 Enamic이 Polyglass보다 유의하게 높은 탄성율을 나타내었으며 (p<0.05), 표면조도가 감소함에 따라 Enamic과 Polyglass 모두 탄성율이 증가하여 E3에서 7.0±0.4 GPa로 최대 탄성율을 나타내었고, P3에서 5.1±0.8 GPa로 최대 탄성율을 나타내었다.
단일 에지 V노치 빔 방법으로 측정한 파괴인성 결과(Table 3)는 표면조도가 동일할 때 Polyglass가 Enamic보다 높은 값을 나타내었으나 유의차는 없었다(p>0.05). 표면조도가 감소함에 따라 Enamic과 Polyglass 모두 파괴인성이 증가하는 음의 상관 관계를 나타내어 Enamic은 E3에서 1.51±0.21 MPa√m로 최대값을 나타내었고, Polyglass는 P3에서 1.84±0.6 MPa√m로 최대 파괴인성을 나타내었다.
와이블 통계분석를 수행하여 굴곡강도를 파괴확률에 따라 도식화한 그래프와 굴곡강도의 와이블 분포를 Figure 4과 Figure 5에 나타내었으며, 각각의 수치를 Table 4에 명시하였다. 파괴확률이 63.21%인 와이블 특성강도는 동일한 표면조도에서 Polyglass가 Enamic보다 높은 값을 나타내었고, 표면조도가 감소함에 따라 파괴확률이 63.21%인 와이블 특성강도가 증가하여 Polyglass는 P3가 139.6 MPa로 최대값을, Enamic은 E3가 122.0 MPa로 최대값을 나타내었다. 또한, 선형회귀분석을 수행하여 기울기로부터 구한 와이블 계수는 Polyglass가 (19.52±0.64)~(22.46±0.89)로 Enamic의 (12.72±0.52)~(19.01±0.67)보다 높은 값을 나타내었다.
총괄 및 고찰
세라믹 재료는 본질적으로 인장력에 취약해서 제작 공정에서 표면에 미세 균열이 생성되기 쉬우며(Chen 등, 1999), 임상 적용 중에 반복적인 하중을 받게 되면 실패율이 증가할 수 있다(Cai 등, 1994; Myers 등, 1994; White 등, 1995). 실제로 임상에서 실패한 대다수의 세라믹 크라운은 교합부위에 초기 균열을 보여서(Anusavice, 1992; Harvey와 Kelly, 1996; Kelly, 1995; Kelly 등, 1990), 제작 과정의 숙련도가 수복물 내구성 확보에 매우 중요한 요인이 된다(Lehner 등, 1997; Proebster, 1996; Scotti 등, 1995). 제작 과정에서 야기되는 문제는 CAD/CAM 시스템이 도입되면서 극복할 수 있게 되어 세라믹 재료는 CEREC (Chairside Economical Restorations of Esthetic Ceramics)으로 대표되는 CAD/CAM 시스템의 중심이 되었다(Shenoy와 Shenoy, 2010).
세라믹-고분자 복합재료는 50여 년 전부터 치과용 컴포짓 레진으로 사용되기 시작했으며, 컴포짓 레진의 물성은 레진 기질, 필러의 종류, 형태 및 크기와 계면 결합제 등에 영향을 받는다. 레진 기질은 초기의 bis-phenol A-glycidyl methacrylate (BisGMA)에서 큰 변화가 없는 반면, 필러는 발전을 거듭하여 컴포짓 레진의 분류의 기준이 되었다(Ferracane, 2011). 컴포짓 레진은 버(bur) 한 세트로 최대 10개의 밀링(milling)이 가능한 세라믹 CAD/CAM 블록에 비해 100개 이상을 치핑(chipping) 없이 가공할 수 있고, 밀링 후 소결할 필요가 없으며, 경도가 낮아서 저작시 대합치의 과다 마모를 방지할 수 있다는 장점이 있어서 CAD/CAM 블록으로도 적용되었다(He와 Swain, 2011; Tsitrou 등, 2007).
3M ESPE는 컴포짓 레진인 Z100을 중합하여 CAD/CAM 블록을 최초로 제작하여 MZ100을 출시한 이후, 필러를 개질한 Lava Ultimate을 레진 나노 세라믹 블록이라고 출시하였다. 국내에서도 유사한 제품으로 베리콤의 Polyglass와 Mazic Duro가 출시되어 현재 임상에서 사용하고 있다. Vita에서는 InCeram과 유사한 방법으로 세라믹-폴리머 블록을 개발하였다. 알루미나 다공체를 만든 후에 치과용 컴포짓 레진의 주성분인 메타아크릴레이트 단량체로 기공을 채우고 중합하였는데 알루미나의 굴절율(1.78)과 메타아크릴레이트 단량체의 굴절율(1.48~1.53)의 차이로 인해 투명성이 좋지 않고, 단량체가 중합하면서 내부에 응력이 발생하여 알루미나와 고분자 간에 debonding이 발생할 수 있다(Mainjot 등, 2011). 그래서 알루미나를 메타아크릴레이트와 굴절율이 비슷한 결정화 유리로 대체하여 투명성을 개선하고, 중합 조건을 조절하여 내부 응력을 감소시켜 Enamic을 하이브리드 세라믹 또는 고분자 침투 세라믹 망목이라고 출시하였다.
컴포짓 레진은 사용 중 파손, 변색 또는 마모되면 손상되지 않은 치아 조직을 경제적으로 보존하면서 수리하거나 교체할 수 있으며(Kallio 등, 2013), 컴포짓 레진 외에도 섬유강화 컴포짓(FRC) 등 고정성 보철물의 수리는 일반적인 임상 술식이 되었다(Freilich 등, 1998; Goldberg와 Burstone, 1992; Kallio 등, 2001; Rosentritt 등, 1998; Sewon 등, 2000; Vallittu, 1999; Vallittu와 Sevelius, 2000).
컴포짓 레진의 물성은 장기적으로 시간에 따라 저하되며(Ferracane, 2006), 이는 레진의 유동도와 소수성에 따른 미세 구조(Sideridou 등, 2003) 및 필러의 조성, 충진율, 비표면적(Kalachandra, 1989)에 의존한다. 컴포짓 레진의 수리를 위해서는 타액에 의해 변화된 표면층을 깨끗이 제거하여 높은 에너지를 갖는 표면을 노출시켜 표면적을 증가시키기 위해 표면 처리한다(Rodrigues Jr. 등, 2009). 표면 처리 후에는 표면 조도가 변하여 컴포짓 레진의 결합력에 영향을 미치게 된다(Bonstein 등, 2005; Bouschlicher 등, 1997).
CAD/CAM으로 제작한 세라믹 수복물은 50~60 μm 입자크기의 다이아몬드 연마 입자가 코팅된 버로 가공해서 초기에 높은 표면 조도를 나타내어(Flurry 등, 2012) 마무리 작업과 연마를 통해 표면 조도를 낮춰야 한다. 표면 조도가 높으면 변색이 증가하고(Yilmaz 등, 2008), 치석 축적이 촉진되며(Bollen 등, 1997; Kawai 등, 2000), 대합치의 마모를 증가시키는(Al-Wahadni와 Martin, 1999; Heintze 등, 2008) 등 일반적으로 세라믹의 강도에 부정적 영향을 미치기 때문이다(De Jager 등, 2000; Fischer 등, 2003; Griggs 등, 1996; Nakamura 등, 2010). 세라믹의 마무리는 표면을 매끄럽게 하고, 대합치의 마모를 줄이는 것이 목적이다(Kou 등, 2006). 세라믹 표면을 연마하면 기계적 특성에 영향을 줄 수 있기 때문에 가공 후에 연마하면 결함 및 균열을 제거하여 강도가 높일 수 있다(Giordano 등, 1995; Guazzato 등, 2005; Johnson-Walls 등, 1986; Williamson 등, 1996). 따라서 접착 후에 수복물을 연마하여 조정하는 것은 임상적으로 관심을 끌어서 다양한 치과용 세라믹의 표면 조도가 연구되었다(Bessing과 Wiktorsson, 1983; Hulterstrom과 Bergman, 1993; Jung, 2002; Martinez-Gomis 등, 2003; Schlissel 등, 1980).
본 연구에서는 세라믹-고분자 CAD/CAM 불록 제품인 Polyglass와 Enamic을 대상으로 절삭가공한 후에 6 μm와 1 μm의 다이아몬드 페이스트로 연마한 3가지의 표면조도를 갖는 시편을 대상으로 기계적 물성을 측정하여 표면조도와 기계적 물성의 관계를 규명하고자 하였다. 기계적 물성은 ISO 국제 표준 규격에 따라 굴곡강도, 와이블 계수 및 특성 강도, 탄성율, 파괴인성을 측정하였다.
시편의 표면을 Atomic Force Microscope으로 관찰하여 최대 거칠기(Rz)와 산술평균 거칠기(Ra)를 측정하였다. 절삭 가공 후 6 μm의 다이아몬드 페이스트로 연마하면 산술평균 거칠기는 46.64~48.62%로 감소하고, 최대 거칠기는 67.80~69.02%로 감소하였다가, 1 μm의 다이아몬드 페이스트로 연마하면 산술평균 거칠기가 12.51~21.61%로 감소하고, 최대 거칠기는 15.94~18.52%로 감소하였다(Table 2).
굴곡강도는 시편을(4.0×1.2×17.0) mm의 크기로 제작한 후, span 길이를 12 mm로 하여 3점 굴곡강도를 측정하였다. 세라믹-고분자 복합재료이므로 소성 변형하여 파괴인성이 증가할 것으로 기대하였으나 Figure 3에 나타낸 응력-변형율 곡선에서 볼 수 있는 바와 같이 Polyglass와 Enamic 모두 응력이 증가함에 따라 변형이 직선적으로 증가하다가 파괴되는 세라믹과 매우 흡사한 거동을 나타내었다. 기울기로부터 계산한 탄성율을 Table 3에 나타내었는데, 모든 표면조도에서 Enamic의 탄성율이 Polyglass보다 유의하게 높았고 (p<0.05), 표면조도가 감소함에 따라 Enamic과 Polyglass 모두 탄성율이 증가하는 음의 상관관계를 나타내어 E3에서 7.0±0.4 GPa와 P3에서 5.1±0.8 GPa로 최대값을 나타내었다. 복합재료의 물성은 분산상보다는 매트릭스에 더 의존하기 때문에 Enamic의 탄성율이 Polyglass보다 높은 것이라고 설명할 수 있다. 본 연구에서 측정한 Enamic과 Polyglass의 탄성율은 모두 자연치(상아질 16.0~20.3 GPa, 법랑질 48.0~105.5 GPa (Coldea 등, 2013))보다 낮았다.
굴곡강도는 모든 표면조도에서 Polyglass가 Enamic보다 2군을 제외하고는 유의하게 높은 굴곡강도를 나타내었다(p<0.05). 모든 표면조도에서 Polyglass는 표면조도가 감소함에 따라 굴곡강도가 증가하는 음의 상관관계를 나타내었으며, 이는 기존에 발표된 연구 보고와도 일치한다(Guazzato 등, 2004; Lohbauer 등, 2008; Nakamura 등, 2010; Sato 등, 2008). 연마하면 표면 조도의 변화 뿐 아니라(Giordano 등, 1995) 표면에 압축 응력과 결함이 발생되어 기계적 물성에 서로 다른 영향을 미친다고 알려져 있다(Pfeiffer와 Hollstein, 1997; Tuan과 Kuo, 1998; Kosmac 등, 1999). 그래서, 연마 후에 표면 조도가 증가하면 굴곡강도가 감소했다는 보고도 있고(Tuan과 Kuo, 1998), 영향이 없다는 보고도 있다(Liao 등, 1997). Polyglass는 P3에서 136.31±7.23 MPa의 최대값을 나타내었으나, Enamic은 E2에서 117.68±7.35 MPa의 최대값을 나타낸 후 E3에서는 비슷한 값을 나타내었는데, 이는 굴곡강도는 표면 조도 뿐 아니라 기공, 미세구조 응력 및 표면과 내부의 결함에 의존하기 때문이라고 생각된다(Albakry 등, 2004; De Jager 등, 2000; Flurry 등, 2012).
세라믹은 외부에서 힘이 가해지면 응력이 결함에 집중되어 파괴가 시작되는 취성 재료이므로 재료에 존재하는 결함에 매우 민감하여 강도의 분포가 넓기 때문에 평균값을 안다고 해도 실제 강도를 예측하기가 어려워서, 일정한 응력에서 파괴가 일어날 확률을 구하는 와이블 통계분석이 세라믹의 신뢰성을 구하는데 널리 사용된다. 와이블 계수는 결함 크기 분포와 관련된 경험 상수로 와이블 계수가 낮을수록 재료의 결함 및 결함이 많고 신뢰성이 떨어지고, 와이블 계수가 크면 결함 밀도가 낮아서 강도의 분포가 작기 때문에 구조적 신뢰성이 높아진다(Albero 등, 2015; Gonzaga 등, 2011; Pittayachawan 등, 2007). 본 연구에서 측정한 와이블 계수는 Polyglass가 19.52±0.64~22.46±0.89로 Enamic의 12.72±0.52~19.01±0.67보다 높았다. 이는 굴곡강도가 높으면 높은 와이블 계수를 나타낸다는 기존의 보고(Guazzato 등, 2005; Kosmac 등, 1999; Rodrigues 등, 2009)와 일치하는 결과이다.
파괴확률이 63.21%인 와이블 특성 강도는 표면조도가 같을 때 Polyglass가 Enamic보다 높은 값을 나타내었고, 표면조도가 감소함에 따라 와이블 특성 강도가 증가하여 Polyglass는 P3가 139.60 MPa로 최대값을, Enamic은 E3가 121.96 MPa로 최대값을 나타내었다.
치과 수복물은 저작 중에 200~1000 N의 교합력이 인가되어 상당한 응력이 발생하여 변형을 일으킬 수 있다. 균열전파에 대한 저항을 파괴인성이라고 정의하며, 취성 재료의 변형 에너지 흡수 능력을 나타내므로(Seghi 등, 1995), 치과수복재료를 임상에서 사용할 때 강도보다 더 중요한 물성이다(Bona 등, 2014). 세라믹의 파괴인성을 측정하는 방법은 단일 에지 V 노치 빔 방법, 쉐브론 노치 빔(chevron notched beam) 방법, 이중 비틀림(double torsion) 방법, 단일 에지선균열 빔(single-edge precracked beam) 방법, 표면 균열굴곡(surface crack in flexure) 방법, 이중 균열 천공 압축(double-cleavage-drilled compression)법, 압입 강도(indentation strength)법, 압입 균열(indentation fracture)법 등 다양하다(Coric 등, 2016; Fischer와 Marx, 2002; Fischer 등, 2008; Gong 등, 2002; Gonzaga 등, 2009, 2011; Kruzic과 Ritchie, 2003; Kruzic 등, 2009; Lawn과 Marshall, 1979; Lee와 Tomozawa, 1999; Lise 등, 2014; Quinn과 Bradt, 2007; Rizkalla와 Jones, 2004; Rocha-Rangel, 2011; Sakar-Deliormanli와 Guden, 2006; Scherrer 등, 1998, 1999; Yana 등, 2006). 시편의 제조와 측정이 용이하여 압입법이 세라믹에서 널리 사용되지만 오차가 커서 부정확하고(Anstis 등, 1981), 단일 에지 V 노치 빔 방법보다 높은 값으로 측정된다고 알려져 있다(Aboushelib 등, 2008; Nawa 등, 1998; Tanaka 등, 2002, 2003).
국제 라운드 로빈(round robin) 평가결과 가장 정확하고 신뢰성이 있으며 재현성이 있어서(ASTM C1421; ISO 23146)국제표준규격에서 추천하는 방법은 단일 에지 V 노치 빔방법이다. 따라서 본 연구에서는 국제표준규격 ISO 6872의 Annex A. Fracture toughness에 따라 시편을(3.0×4.0×17.0) mm의 크기로 제작한 후, span 길이를 12 mm로 하여 단일 에지 V 노치 빔 방법으로 파괴인성을 측정하였다. 다이아몬드 휠과 페이스트를 이용하여 깊이가 0.8~1.2 mm이고, 곡률반경이 30° 이하인 V 노치를 만든 후, 노치가 아래쪽을 향하도록 span 길이 12 mm의 지지대위에 올려놓고, 0.5 mm/min의 속도로 하중을 가하였다. 본 연구에서 측정한 파괴인성은 표면조도가 동일할 때 Polyglass가 Enamic보다 높았으나 유의한 차이는 없었고 (p>0.05), 표면조도가 감소함에 따라 Enamic과 Polyglass 모두 파괴인성이 증가하는 음의 상관관계를 나타내어 Enamic은 E3에서 1.51±0.21 MPa√m로 최대값을 나타내었고, Polyglass는 P3에서 1.84±0.6 MPa√m로 최대 파괴인성을 나타내었다. 본 연구에서 측정한 Enamic과 Polyglass의 파괴인성은 법랑질(0.7~1.37 MPa√m (Vieira 등, 2012), 0.6~1.5 MPa√m (Coldea 등, 2013))과 유사하였다.
결 론
치과용 CAD/CAM 세라믹-고분자 블록인 Polyglass와 Enamic의 표면조도를 변화시키며 기계적 물성을 측정한 결과 다음과 같은 결과를 얻었다.
- 1. 굴곡 하중을 가하면 Polyglass와 Enamic 모두 직선적으로 증가하다가 파괴되어, 소성 변형은 거의 발생하지 않음을 알 수 있었다.
- 2. Polyglass와 Enamic 모두 표면조도가 감소함에 따라 굴곡강도, 와이블 계수, 탄성율, 파괴인성은 증가하는 음의 상관관계를 나타내었다.
- 3. Polyglass의 기계적 물성은 P3까지 계속 증가하였으나, Enamic은 E2에서 거의 최대값을 나타내어 E2와 E3간에 유의차가 없었다.
따라서 고분자 매트릭스 구조에 세라믹 입자가 분산강화 된 Polyglass는 표면조도가 낮을수록 기계적 물성이 증가하지만, 세라믹 매트릭스에 고분자가 함침된 Enamic은 6 μm의 다이아몬드 페이스트로 연마하면 기계적 물성의 최대값을 나타내기에 충분하였음을 알 수 있었다.
이는 본 실험에 사용한 두 가지 재료의 구조적 차이에 기인한 것으로 생각되나, 세라믹과 고분자의 조성 뿐 아니라 함량과 크기도 달라서 명확한 결론을 위해서는 계속적인 연구가 필요하다고 생각된다.
Acknowledgments
* 본 논문은 2017년도 조선대학교 지원을 받았음.
참고문헌
- 박종환, 치과수복용 CAD/CAM 세라믹/고분자 블록의 기계적 물성, (2017), 조선대학교 박사학위논문.
- 3M Paradigm, (2011), Lava Ultimate. CAD/CAM Block, Technical product profile.
- 3M Paradigm, (2000), MZ100 Block, Technical product profile.
-
Al-Wahadni, AM, Martin, DM, (1999), An in vitro investigation into the wear effects of glazed, unglazed and refinished dental porcelain on an opposing material, J Oral Rehabil, 26(6), p538-546.
[https://doi.org/10.1046/j.1365-2842.1999.00394.x]
-
Albakry, M, Guazzato, M, Swain, MV, (2003), Biaxial flexural strength, elastic moduli, and x-ray diffraction characterization of three pressable all-ceramic materials, J Prosthet Dent, 89(4), p374-380.
[https://doi.org/10.1067/mpr.2003.42]
-
Albakry, M, Guazzato, M, Swain, MV, (2004), Effect of sandblasting, grinding, polishing and glazing on the flexural strength of two pressable all-ceramic dental materials, J Dent, 32(2), p91-99.
[https://doi.org/10.1016/j.jdent.2003.08.006]
-
Anstis, GR, Chantikul, P, Lawn, BR, Marshall, DB, (1981), A critical evaluation of indentation techniques for measuring fracture toughness: I, Direct crack measurements, J Am Ceram Soc, 64(9), p533-538.
[https://doi.org/10.1111/j.1151-2916.1981.tb10320.x]
-
Anusavice, KJ, Degradability of dental ceramics, (1992), Adv Dent Res, 6, p82-9.
[https://doi.org/10.1177/08959374920060012201]
- ASTM C1421:16 Standard test methods for determination of fracture toughness of advanced ceramics at ambient temperature.
-
Bagheri, R, Tyas, MJ, Burrow, MF, (2007), Subsurface degradation of resin-based composites, Dent Mater, 23(8), p944-951.
[https://doi.org/10.1016/j.dental.2006.06.035]
-
Bessing, C, Wiktorsson, A, (1983), Comparison of two different methods of polishing porcelain, Scand J Dent Res, 91, p482-487.
[https://doi.org/10.1111/j.1600-0722.1983.tb00849.x]
- Bollen, CM, Lambrechts, P, Quirynen, M, (1997), Comparison of surface roughness of oral hard materials to the threshold surface roughness for bacterial plaque retention: A review of the literature, Dent Mater, 13(4), p258-269.
- Bona, AD, Corazza, PH, Zhang, Y, (2014), Characterization of a polymer-infiltrated ceramic-network material, Dent Mater, 30, p564-569.
- Bonstein, T, Garlapo, D, Donarummo, J, Bush, PJ, (2005), Evaluation of varied repair protocols applied to aged composite resin, J Adhes Dent, 7, p41-49.
- Bouschlicher, MR, Reinhardt, JW, Vargas, MA, (1997), Surface treatment techniques for resin composite repair, Am J Dent, 10, p279-283.
-
Brewer, JD, Garlapo, DA, Chipps, EA, Tedesco, LA, (1990), Clinical discrimination between autoglazed and polished porcelain surfaces, J Prosthet Dent, 64(6), p631-635.
[https://doi.org/10.1016/0022-3913(90)90284-j]
-
Cai, H, Kalceff, MA, Lawn, BR, (1994), Deformation and fracture of mica-containing glass-ceramics in Hertzian contacts, J Mater Res, 9, p762-770.
[https://doi.org/10.1557/jmr.1994.0762]
-
Chen, HY, Hickel, R, Setcos, JC, Kunzelmann, KH, (1999), Effects of surface finish and fatigue testing on the fracture strength of CAD-CAM and pressed-ceramic crowns, J Prosthet Dent, 82(4), p468-475.
[https://doi.org/10.1016/s0022-3913(99)70036-3]
- Chu, FC, Frankel, N, Smales, RJ, (2000), Surface roughness and flexural strength of self-glazed, polished and reglazed In-Ceram/Vitadur Alpha porcelain laminates, Inter J Prosthodont, 13(1), p66-71.
-
Coldea, A, Swain, MV, Thiel, N, (2013), In-vitro strength degradation of dental ceramics and novel PICN material by sharp indentation, J Mech Behav Biomed Mater, 26, p34-42.
[https://doi.org/10.1016/j.jmbbm.2013.05.004]
-
Coldea, A, Swain, MV, Thiel, N, (2013), Mechanical properties of polymer-infiltrated- ceramic-network materials, Dent Mater, 29, p419-426.
[https://doi.org/10.1016/j.dental.2013.01.002]
- Coric, D, Remjo, MM, Curkovic, L, Zmak, I, (2016), Indentation fracture toughness of Y-TZP dental ceramics, 16th International Conference on New Trends in Fatigue and Fracture, 1-6, 5. 24-27, Croatia.
-
De Jager, N, Feilzer, AJ, Davidson, CL, (2000), The influence of surface roughness on porcelain strength, Dent Mater, 16(6), p381-388.
[https://doi.org/10.1016/s0109-5641(00)00030-0]
-
Ferracane, JL, Elution of leachable components from composites, (1994), J Oral Rehabil, 21(4), p441-452.
[https://doi.org/10.1111/j.1365-2842.1994.tb01158.x]
-
Ferracane, JL, (2006), Hygroscopic and hydrolytic effects in dental polymer networks, Dent Mater, 22(3), p211-222.
[https://doi.org/10.1016/j.dental.2005.05.005]
-
Fischer, H, Marx, R, (2002), Fracture toughness of dental ceramics: Comparison of bending and indentation method, Dent Mater, 18, p12-19.
[https://doi.org/10.1016/s0109-5641(01)00005-7]
-
Fischer, H, Schafer, M, Marx, R, (2003), Effect of surface roughness on flexural strength of veneer ceramics, J Dent Res, 82(12), p972-975.
[https://doi.org/10.1177/154405910308201207]
-
Fischer, H, Waindich, A, Telle, R, (2008), Influence of preparation of ceramic SEVNB specimens on fracture toughness testing results, Dent Mater, 24, p618-622.
[https://doi.org/10.1016/j.dental.2007.06.021]
- Freilich, MA, Duncan, JP, Meiers, JC, Goldberg, AJ, (1998), Preimpregnated fiber- reinforced prostheses. Part I. Basic rationale and complete coverage and intracoronal fixed partial denture designs, Quintessence Int, 29, p689-696.
- Fuzzi, M, Zaccheroni, Z, Vallania, G, (1996), Scanning electron microscopy and profilometer evaluation of glazed and polished dental porcelain, Inter J Prosthodont, 9(5), p452-458.
- Giordano, R, Cima, M, Pober, R, (1995), Effect of surface finish on the flexural strength of feldspathic and aluminous dental ceramics, Int J Prosthodont, 8, p311-319.
-
Goldberg, AJ, Burstone, CJ, (1992), The use of continuous fiber reinforcement in dentistry, Dent Mater, 8, p197-202.
[https://doi.org/10.1016/0109-5641(92)90083-o]
- Gong, J, Wang, J, Guan, Z, (2002), Indentation toughness of ceramics: A modified approach, J Mater Sci, p865-869.
-
Gonzaga, CC, Cesar, PF, Miranda, Jr. WJ, Yoshimura, HN, (2011), Slow crack growth and reliability of dental ceramics, Dent Mater, 27, p394-406.
[https://doi.org/10.1016/j.dental.2010.10.025]
-
Griggs, JA, Thompson, JY, Anusavice, KJ, (1996), Effects of flaw size and auto-glaze treatment on porcelain strength, J Dent Res, 75(6), p1414-1417.
[https://doi.org/10.1177/00220345960750061301]
- Groger, G, Rosentritt, M, Behr, M, Schroder, J, Handel, G, (2006), Dental resin materials in vivo - TEM results after one year: A pilot study, J Mater Sci Mater Med, 17(9), p825-828.
-
Guazzato, M, Albakry, M, Quach, L, Swain, MV, (2004), Influence of grinding, sand- blasting, polishing and heat treatment on the flexural strength of a glass-infiltrated alumina-reinforced dental ceramic, Biomaterials, 25(11), p2153-2160.
[https://doi.org/10.1016/j.biomaterials.2003.08.056]
-
Guazzato, M, Albakry, M, Quach, L, Swain, MV, (2005), Influence of surface and heat treatments on the flexural strength of a glass-infiltrated alumina/ zirconiareinforced dental ceramic, Dent Mater, 21(5), p454-463.
[https://doi.org/10.1016/j.dental.2004.07.010]
-
Harvey, CK, Kelly, JR, (1996), Contact damage as a failure mode during in vitro testing, J Prosthodont, 5, p95-100.
[https://doi.org/10.1111/j.1532-849x.1996.tb00281.x]
-
He, LH, Swain, M, (2011), A novel polymer infiltrated ceramic dental material, Dent Mater, 27, p527-534.
[https://doi.org/10.1016/j.dental.2011.02.002]
-
Heintze, SD, Cavalleri, A, Forjanic, M, Zellweger, G, Rousson, V, (2008), Wear of ceramic and antagonist – a systematic evaluation of influencing factors in vitro, Dent Mater, 24(4), p433-449.
[https://doi.org/10.1016/j.dental.2007.06.016]
- Hulterstrom, AK, Bergman, M, (1993), Polishing systems for dental ceramics, Acta Odontol Scand, 51, p229-234.
- ISO 6872:2015 Dentistry - Ceramic materials.
- ISO 13061-4:2014 Physical and mechanical properties of wood - Test methods for small clear wood specimens - Part 4: Determination of modulus of elasticity in static bending.
-
Jaffer, F, Finer, Y, Santerre, JP, (2002), Interactions between resin monomers and commercial composite resins with human saliva derived esterases, Biomaterials, 23(7), p1707-1719.
[https://doi.org/10.1016/s0142-9612(01)00298-8]
-
Johnson-Walls, D, Evans, AG, Marshall, BD, James, MR, (1986), Residual stresses in machined ceramic surfaces, J Am Ceram Soc, 69, p44-47.
[https://doi.org/10.1111/j.1151-2916.1986.tb04691.x]
- Jung, M, (2002), Finishing and polishing of a hybrid composite and a heat-pressed glass ceramic, Oper Dent, 27, p175-183.
-
Kalachandra, S, (1989), Influence of fillers on the water sorption of composites, Dent Mater, 5, p283-288.
[https://doi.org/10.1016/0109-5641(89)90077-8]
-
Kallio, TT, Lastumaki, TM, Vallittu, PK, (2001), Bonding of restorative and veneering composite resin to some polymeric composites, Dent Mater, 17, p80-86.
[https://doi.org/10.1016/s0109-5641(00)00064-6]
-
Kallio, TT, Tezvergil-Mutluay, A, Lassila, LV, Vallittu, PK, (2013), The effect of surface roughness on repair bond strength of light-curing composite resin to polymer composite substrate, Open Dent J, 7, p126-131.
[https://doi.org/10.2174/1874210601307010126]
- Kawai, K, Urano, M, Ebisu, S, (2000), Effect of surface roughness of porcelain on adhesion of bacteria and their synthesizing glucans, J Prosthet Dent, 83(6), p664-667.
-
Kelly, JR, (1995), Perspectives on strength, Dent Mater, 11, p103-110.
[https://doi.org/10.1016/0109-5641(95)80043-3]
- Kelly, JR, Giordano, R, Pober, R, Cima, MJ, (1990), Fracture surface analysis of dental ceramics: clinically failed restorations, Int J Prosthodont, 3, p430-440.
- Kosmac, T, Oblak, C, Jevnikar, P, Funduk, N, Marion, L, (1999), The effect of surface grinding and sandblasting on flexural strength and reliability of Y-TZP zirconia ceramic, Dent Mater, 15, p426-433.
-
Kou, W, Molin, M, Sjogren, G, (2006), Surface roughness of five different dental ceramic core materials after grinding and polishing, J Oral Rehabil, 33(2), p117-124.
[https://doi.org/10.1111/j.1365-2842.2006.01546.x]
-
Kruzic, JJ, Ritchie, RO, (2003), Determining the toughness of ceramics from Vickers indentations using the crack-opening displacements: An experimental study, J Am Ceram Soc, 86(8), p1433-1436.
[https://doi.org/10.1111/j.1151-2916.2003.tb03490.x]
-
Kruzic, JJ, Kim, DK, Koester, KJ, Ritchie, RO, (2009), Indentation techniques for evaluating the fracture toughness of biomaterials and hard tissues, J Mech Behav Biomed, 2(4), p384-395.
[https://doi.org/10.1016/j.jmbbm.2008.10.008]
-
Lawn, BR, Marshall, DB, (1979), Hardness, toughness, and brittleness: An indentation analysis, J Am Ceram Soc, 62(7-8), p347-350.
[https://doi.org/10.1111/j.1151-2916.1979.tb19075.x]
- Lee, YK, Tomozawa, M, (1999), Effect of water content in phosphate glasses on crack growth rate, J Non-Cryst Solids, 248, p203-210.
-
Lehner, C, Studer, S, Brodbeck, U, Scharer, P, (1997), Short-term results of IPS Empress full-porcelain crowns, J Prosthodont, 6, p20-30.
[https://doi.org/10.1111/j.1532-849x.1997.tb00061.x]
-
Liao, TW, Li, K, Breder, K, (1997), Flexural strength of ceramics ground under widely different conditions, J Mater Process Tech, 70, p198-206.
[https://doi.org/10.1016/s0924-0136(97)80801-1]
- Liu, PR, (2005), A panorama of dental CAD/CAM restorative systems, Compendium, 26(7), p507-513.
-
Lohbauer, U, Kramer, N, Petschelt, A, Frankenberger, R, (2008), Correlation of in vitro fatigue data and in vivo clinical performance of a glassceramic material, Dent Mater, 24(1), p39-44.
[https://doi.org/10.1016/j.dental.2007.01.011]
-
Lohbauer, U, Muller, FA, Petschelt, A, (2008), Influence of surface roughness on mechanical strength of resin composite versus glass ceramic materials, Dent Mater, 24(2), p250-256.
[https://doi.org/10.1016/j.dental.2007.05.006]
-
Mainjot, AK, Schajer, GS, Vanheusden, AJ, Sadoun, MJ, (2011), Residual stress measurement in veneering ceramic by hole-drilling, Dent Mater, 27(5), p439-444.
[https://doi.org/10.1016/j.dental.2010.12.002]
- Martinez-Gomis, J, Bizar, J, Anglada, JM, Samso, J, Peraire, M, (2003), Comparative evaluation of four finishing systems on one ceramic surface, Int J Prosthodont, 16, p74-77.
- Miyazaki, T, Hotta, Y, Kunii, J, Kuriyama, S, Tamaki, Y, (2009), A review of dental CAD/ CAM: Current status and fracture perspectives from 20 years of experience, Dent Mater J, 28(1), p44-56.
- Myers, ML, Engle, JW, Fairhurst, CW, Ringle, RD, (1994), Fatigue failure parameters of IPS–Empress porcelain, Int J Prosthodont, 7, p549-753.
-
Nakamura, Y, Satoru, H, (2010), The effect of surface roughness on the Weibull distribution of porcelain strength, Dent Mater J, 29(1), p30-34.
[https://doi.org/10.4012/dmj.2009-059]
-
Nawa, M, Nakamoto, S, Sekino, T, Niihara, K, (1998), Tough and strong Ce-TZP/alumina nanocomposites doped with titania, Ceram Inter, 24(7), p497-506.
[https://doi.org/10.1016/s0272-8842(97)00048-5]
- Nguyen, JF, Migonney, V, Ruse, ND, Sadoun, M, (2012), Resin composite blocks via high- temperature polymerization, Dent Mater, 28, p529-534.
- Nguyen, JF, Ruse, D, Phan, AC, Sadoun, MJ, (2014), High-temperature-pressure polymerized resin-infiltrated ceramic network, J Dent Res, 91(3), p62-67.
-
Ortengren, U, Wellendorf, H, Karlsson, S, Ruyter, I, (2001), Water sorption and solubility of dental composites and identification of monomers released in an aqueous environment, J Oral Rehabil, 28(12), p1106-1115.
[https://doi.org/10.1046/j.1365-2842.2001.00802.x]
- Pfeiffer, W, Hollstein, T, (1997), Characterisation and assessment of machined ceramic surfaces, Interceram, 146, p98-102.
-
Pittayachawan, P, McDonald, A, Petrie, A, Knowles, JC, (2007), The biaxial flexural strength and fatigue property of Lava™ Y-TZP dental ceramic, Dent Mater, 23(8), p1018-1029.
[https://doi.org/10.1016/j.dental.2006.09.003]
- Proebster, L, (1996), Four year clinical study of glass-infiltrated, sintered alumina crowns, J Oral Rehabil, 23, p147-151.
-
Quinn, GD, Bradt, RC, (2007), On the Vickers indentation fracture toughness test, J Am Ceram Soc, 90(3), p673-680.
[https://doi.org/10.1111/j.1551-2916.2006.01482.x]
- Rocha-Rangel, E, (2011), Fracture toughness determinations by means of indentation fracture, p21-38, in Nanocomposites with Unique Properties and Applications in Medicine and Industry, Edited by Cuppolett, J, Intech.
-
Rosentritt, M, Behr, M, Leibrock, A, Handel, G, Friedl, K, (1998), Intraoral repair of fiber-reinforced composite fixed partial dentures, J Prosthet Dent, 79, p393-398.
[https://doi.org/10.1016/s0022-3913(98)70151-9]
- Sakar-Deliormanli, A, Guden, M, (2006), Microhardness and fracture toughness of dental materials by indentation method, J Biomed Mater Res Part B. Appl Biomater, 76B(2), p257-264.
-
Sato, H, Yamada, K, Pezzotti, G, Nawa, M, Ban, S, (2008), Mechanical properties of dental zirconia ceramics changed with sandblasting and heat treatment, Dent Mater J, 27(3), p408-414.
[https://doi.org/10.4012/dmj.27.408]
-
Scherrer, SS, Denry, IL, Wiskott, HW, (1998), Comparison of three fracture toughness testing techniques using a dental glass and a dental ceramic, Dent Mater, 14, p246-255.
[https://doi.org/10.1016/s0109-5641(98)00032-3]
-
Schlissel, ER, Newitter, DA, Renner, RR, Gwinnett, AJ, (1980), An evaluation of postadjustment polishing techniques for porcelain denture teeth, J Prosthet Dent, 43, p258-265.
[https://doi.org/10.1016/0022-3913(80)90399-6]
- Scotti, R, Catapano, S, D’Elia, A, (1995), A clinical evaluation of In-Ceram crowns, Int J Prosthodont, 8, p320-323.
-
Sewon, LA, Ampula, L, Vallittu, PK, (2000), Rehabilitation of a periodontal patient with rapidly progressing marginal alveolar bone loss: 1-year follow-up, J Clin Periodontol, 27, p615-619.
[https://doi.org/10.1034/j.1600-051x.2000.027008615.x]
-
Shenoy, A, Shenoy, N, (2010), Dental ceramics: An update, J Conserv Dent, 13(4), p195-204.
[https://doi.org/10.4103/0972-0707.73379]
- Sherrer, SS, Kelly, JR, Quinn, GD, Xu, K, (1999), Fracture toughness (KIC) of a dental porcelain determined by fractographic analysis, Dent Mater, 15, p342-348.
-
Sideridou, I, Tserki, V, Papanastasiou, G, (2003), Study of water sorption, solubility and modulus of elasticity of light-cured dimethacrylate-based dental resins, Biomaterials, 24, p655-665.
[https://doi.org/10.1016/s0142-9612(02)00380-0]
-
Spitznagel, FA, Horvath, SD, Guess, PC, Blatz, MB, (2014), Resin bond to indirect composite and new ceramic/polymer materials: A review of the literature, J Esthet Restor Dent, 26, p382-393.
[https://doi.org/10.1111/jerd.12100]
-
Tanaka, K, Tamura, J, Kawanabe, K, Nawa, M, Oka, M, Uchida, M, Kokubo, T, Nakamura, T, (2002), Ce‐TZP/Al2O3 nanocomposite as a bearing material in total joint replacement, J Biomed Mater Res, 63(3), p262-270.
[https://doi.org/10.1002/jbm.10182]
-
Tholt, B, Miranda-Junior, WG, Prioli, R, Thompson, J, Oda, M, (2006), Surface roughness in ceramics with different finishing techniques using atomic force micro- scope and profilometer, Oper Dent, 31(4), p442-449.
[https://doi.org/10.2341/05-54]
-
Tsitrou, EA, Northeast, SE, van Noort, R, (2007), Brittleness index of machinable dental materials and its relation to the marginal chipping factor, J Dent, 35, p897-902.
[https://doi.org/10.1016/j.jdent.2007.07.002]
-
Tuan, WH, Kuo, JC, (1998), Effect of abrasive grinding on the strength and reliability of alumina, J Eur Ceram Soc, 18, p799-806.
[https://doi.org/10.1016/s0955-2219(97)00202-1]
-
Vallittu, PK, (1999), Prosthodontic treatment with a glass fiber-reinforced resin- bonded fixed partial denture: A clinical report, J Prosthet Dent, 82, p132-135.
[https://doi.org/10.1016/s0022-3913(99)70145-9]
-
Vallittu, PK, Sevelius, C, (2000), Resin-bonded, glass fiber-reinforced composite fixed partial dentures: a clinical study, J Prosthet Dent, 84, p413-418.
[https://doi.org/10.1067/mpr.2000.109782]
- Vita Enamic, (2012), Technical and scientific documentation.
- Vita Enamic, (2013), The concept.
-
White, SN, (1993), Mechanical fatigue of a feldspathic dental porcelain, Dent Mater, 9(4), p260-264.
[https://doi.org/10.1016/0109-5641(93)90071-w]
- White, SN, Zhao, XY, Zhaokun, Y, Li, ZC, (1995), Cyclic mechanical fatigue of a felds- pathic dental porcelain, Int J Prosthodont, 8, p413-420.
- Williamson, RT, Kovarik, RE, Mitchell, RJ, (1996), Effects of grinding, polishing, and overglazing on the flexure strength of a high leucite feldspathic porcelain, Int J Prosthodont, 9, p30-37.
-
Wright, MD, Masri, R, Driscoll, CF, Romberg, E, Thompson, GA, Runyan, DA, (2004), Comparison of three systems for the polishing of an ultra-low fusing dental porcelain, J Prosthet Dent, 92(5), p486-490.
[https://doi.org/10.1016/j.prosdent.2004.07.021]
- Yana, J, Clifton, KB, Mecholsky, Jr JJ, Reep, RL, (2006), Fracture toughness of manatee rib and bovine femur using a chevron-notched beam test, J Biomech, 39, p1066-1074.
- Yilmaz, C, Korkmaz, T, Demirkoprulu, H, Ergun, G, Ozkan, Y, (2008), Color stability of glazed and polished dental porcelains, J Prosthodont, 17(1), p20-24.
-
Zahran, M, El-Mowafy, O, Tam, L, Watson, PA, Finer, Y, (2008), Fracture strength and fatigue resistance of all-ceramic molar crowns manufactured with CAD/CAM technology, J Prosthodont, 17, p370-377.
[https://doi.org/10.1111/j.1532-849x.2008.00305.x]