
Antifungal effect and characterization of denture PMMA-nanocomposite containing gold, platinum and silver nanoparticles
This study assessed the antifungal effect of modified denture acrylic resin containing Au, Pt and Ag nanoparticles (NPs) respectively and evaluated their physical aspects to produce a antimicrobial nanocomposite material. Each colloidal NPs suspension was preliminary combined to the denture powder in different doses (0, 10, 50, 100 and 200 ppm) then mixed with monomer. The antifungal (C. albicans) effect was assessed using a fluorescence activated cell sorting, thermal analysis and color stability test were conducted to characterize those nanocomposites. As compared to control (0 ppm), the significant reductions of viable cell adherence on the nanocomposite were shown at the dose of 200 ppm in all NPs and 100 ppm in AgNPs. Addition of NPs to PMMA resulted in successful co-polymerization phase with stable thermal property, however, lack of color stability should be improved.
Keywords:
Antifungal effect, Nanoparticles, Noble metal, PMMA denture acrylic resinINTRODUCTION
PMMA (Polymethyl methacrylate) - based resins are widely used as dental materials especially in denture base. However, denture seated in the oral cavity inevitably leads to changes in environmental conditions, compromising the mechanical cleansing effect of salivary flow and encouraging formation of biofilms on both prosthetics and adjacent mucosa (Yildirim et al., 2005). Chronic denture stomatitis is a representative pathogenic condition of the denture-bearing mucosa and one of its major etiologic factors is the yeasts, usually Candida albicans (C. albicans) on the fitting surface of the denture (Vanden Abbeele et al., 2008). Fungal adherence to inert polymer such as PMMA is regarded to be an essential prerequisite for colonization (Klotz et al., 1985), roughness and voids of denture surface could accelerate initial adhesion as well. Despite the use of antifungal drugs to treat denture stomatitis, infection is often persistent or resistant (Chandra et al., 2001). Recently, induction of antimicrobial action in dental materials has been a huge concern and denture acrylic that can strongly resist microbial adhesion is highly requested (Saito et al., 1997), but it has not been available in market yet.
Nanoparticles (NPs), under 100 nm in size, have been studied widely due to their unique physico-chemical characteristics with higher catalytic and antimicrobial activity compared to bulk metal (Park et al., 2011). Particularly, noble nano-sized metals such as gold nano-particles (AuNPs), platinum nanoparticles (PtNPs) and silver nanoparticles (AgNPs) have elicited lots of interest for biomedical applications because of their ease of synthesis, surface functionalization and toxic effects to bacteria and fungi (Chwalibog et al., 2010). The biocidal actions of these NPs were reported as they interact directly with various microbes (Panácek et al., 2009; Sawosz et al., 2010) and they are exploring as an alternative antimicrobial agent (Sondi & Salopek-Sondi, 2004; Lima et al., 2013).
Nanocomposites (NC) are defined as the materials whose major component is a polymer and the minor one must have a single dimension below 100 nm. NC have become an active field of study because of large property changes with very small addition of nanofiller less than 5.0 weight % (Motaung et al., 2012). Polymeric materials such as PMMA denture acrylic offers superior macroscopic properties and is readily processible, it could be an excellent candidate for the formulation of NC based on the inclusion of NPs. Metal-PMMA NC, a typical example of using metal NPs as additives in polymer matrix, was reported to improve the mechanical properties of polymers or antibacterial activity (Sodagar et al., 2012; Boomi et al., 2013). Studies for dental polymers containing NPs have focused on Ag exclusively (Ahn et al., 2009; Wady et al., 2012; Monteiro et al., 2012), though warning for Ag, a concentration-dependent toxicity, necrosis or apoptosis has been demonstrated (Braydich-Stolle et al., 2005), challenging the experiment for another noble metal NPs such as Au or Pt may be needed.
The biocidal activity of Au, Pt and Ag NPs against various bacteria or fungi were known, but few information of efficacy after their incorporation to denture polymer has been reported yet, moreover, NPs could act as impurities which change the physical properties in polymers (Davies & Rawlings, 1999) along with varied chemical interaction between the C=O groups or color stability related with metal particles.
The present study comparatively evaluated the antifungal activity of nanocomposite, a modified denture acrylic impregnated by NPs of Au, Pt and Ag in vitro, then simultaneously assessed the thermal analysis and color stability for mechanical characters meeting those specified for denture bases in clinical situation.
MATERIALS AND METHODS
1. Preparation of nanoparticles
NPs of Au, Pt and Ag were synthesized by chemical reduction method as follows (Figure 1). Each NPs was prepared by mixing the following two solutions in a homomixer (Homomixer Mark II, Tokushu Kika Kogyo Co., Japan). Solution 1 (250 mL) were respectively prepared by dissolving 6.0 g of PVP (Polyvinyl Pyrrolidon) in a aqueous solution of hydrogen tetrachloroaurate (III) (1908 ppm) for AuNPs, chloroplatinic acid hydrate (1908 ppm) for PtNPs and silver nitrate (1908 ppm) for AgNPs . Solution 2 was prepared by dissolving sodium borohydride (0.02 g) in an aqueous solution (250 mL) of PVP (12.5 g). Then, solution 1 and 2 were rapidly mixed by homomixer and the mixture was homogenized at the same speed (3500 rpm) according to the formulation for 5 minutes. All chemical reagents were used without further purification and electron transmission micrograph (TEM; Hitachi H 7100, Tokyo, Japan) of each NPs showed spherically shaped particles under 10 nm in a size (Figure 2).
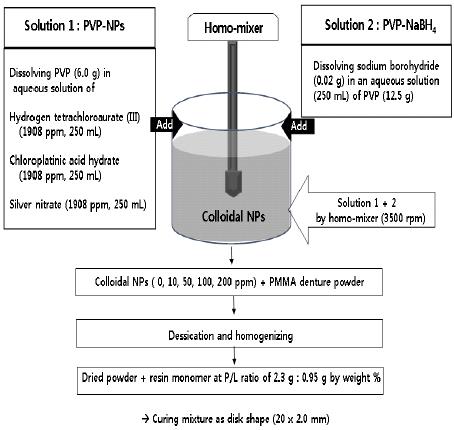
Schematic diagram for preparation of noble metal (Au, Pt, Ag) nanoparticles and fabrication of nanocomposite sample.
2. Fabrication of specimens; metal-nanocomposite (Au-NC, Pt-NC, Ag-NC)
A heat cured denture base acrylic resin (Vertex®, Vertex-Dental B.V., Netherlands) was used and the amounts of NPs loading to PMMA was controlled under 2.0 weight (wt) % maximally to secure the mechanical aspects. Colloidal AuNPs, PtNPs and AgNPs were preliminary combined with the pristine denture powder respectively at the concentrations of 10, 50, 100 and 200 ppm (0.1 ~ 2.0 wt %). Specimen without NPs incorporated (0 ppm) was used as control. Dehydrations of each combined powders were conducted through drying for 120 hours at room temperature and desiccated in an oven at 37 oC for 48 hours. NPs - combined Powders were mixed with resin monomer at designated P/L ratio (2.3 g : 0.95 g by weight %) and the dough-staged mixture was packed into a custom-made brass moulds with disk hole (20. 0 mm x 2.0 mm) then covered by two glass frames with 10 Kg static pressure. All of mixtures were cured following the manufacturer's instructions and trimmed. 260 specimens were fabricated and distributed to each groups (n = 20). They were immersed in sterilized distilled water for 30 days to leach excess residual monomer then cleaned ultrasonically in distilled water with ultrasonic device (Branson 2200; Branson, Danbury, CT, USA).
3.Antifungal assay by fluorescence activated cell sorting (FACS)
C. albicans strain (KCTC 7965) was obtained as a stock culture and antifungal effect was evaluated through FACS with bacterial viability and counting kit (Molecular Probes, Oregon. USA). Prior to C. albicans contamination, NC samples (n = 10) were sterilized with ethylene oxide gas for 24 hours to ensure their initial sterility. To mimic the oral cavity, specimens were coated with synthetic saliva (Taliva, Hanlim Co., South Korea) for 1 hour before incubation with fungi. Fungal seed suspensions (1.0 volume %) to 1.5 mL medium were inoculated on specimens located in 12-well culture plate (Costa, Corning Co., New York, USA), then cultured for 24 hours at 37℃ incubator. Samples were centrifuged at 10,000 g for 3 minutes to pellet the cells followed by washing and sonication with PBS (Phosphate Buffered Saline). Cells were resuspended in 500 µL of PBS which contain 0.1 µL of 3.34 mM SYTO 9 nucleic acid stain (for live and dead fungal staining) and 0.1 µL of 30 mM propidium iodide (PI, for dead fungal-specific staining) and they were incubated for 15 minutes at room temperature protected from light. For the final step, added 1µL of the microsphere suspension to the stained cell sample as a reference standard for sample volume then mixed well and analyzed by flow cytometry analysis (Accuri Flow Cytometer, BD Biosciences, San Jose, USA). Live and dead fungal cells were calculated from either the fluorescence versus side scatter cytogram or the green fluorescence versus red fluorescence cytogram. To confirm the differentiation between live and dead fungi, fluorescent stained images were analyzed under fluorescent microscope (Olympus BX 51, Tokyo, Japan).
4. Thermal analysis
To evaluate the physical property of denture acrylic influenced by incorporating NPs, thermogravimetric (TG) and differential scanning calorimetry (DSC) were carried out simultaneously using a TG-DTA 92 (Setaram, France) instrument with heating rate of 10°C min-1 from 30 to 600 °C under nitrogen atmosphere. Disc Samples were fabricated as described previously, NC samples with the highest NPs dose (200 ppm) and pristine denture acrylic sample (control; 0 ppm) were tested (n=5).
5. Color measurement
Color was measured on the surface of disc sample (20. 0 mm x 2.0 mm) at the time of 120 hours from the onset of curing process (n = 5) and measured as follows; they were made in 3 randomly selected areas by using colorimeter (Color-readerⓇ, Minolta CR-10, Japan) in reflectance mode against the white background of an opacity card. The color is expressed as L*, a* and b*, where L* denotes the lightness, a* denotes the red/green value and b* denotes the yellow/ blue value for measurement. The mean of 3 readings was recorded and the value of each specimen was calculated with the CIE LAB scale recommended by Commission Internationale de l'Eclairage (Polyzois et al,, 1997). Means were calculated for each evaluation and the total color change (ΔE*) of each NC was then calculated using the followed relationship:
6. Statistical analysis
Data were analyzed by a one-way ANOVA with Tukey's HSD multiple comparison tests at the significance level of 0.01.
RESULTS
Antifungal effect of NC at 24 hour-incubation
Effects were determined according to the viable cell counting from biofilms in retrieved suspensions then calculated as the percentage of the control (Table 1 & Figure 3). Most of attached fungal cells were viable while dead cells were detected less than 10 % from total attached cells (Table 1) presented by fluorescent signal specific to live and dead cells staining. When compared to control, all of NC groups with 200 ppm NPs loaded significantly reduced viable fungal adhesion by the rate of -61.0 ± 6.7 % in Ag-NC, -58.6 ± 8.7 % in Pt-NC and -56.6 ± 8.3 % in Au-NC respectively (p < 0.01) but there were no statistical differences among them (p > 0.01). Within 100 ppm NPs loaded group, Ag-NC solely exhibited significant anti-adherent effect by -45.4 ± 6.6 % as compared to control, but there were no statistical differences with Au-NC and Pt-NC (p > 0.01).

Adherence of viable and dead fungal cell on the each NC group according to the different doses of NPs loading through FACS analysis
Thermal stability
Despite the adding of NPs, three NC exhibited similar thermograms to that of control at the experimental temperature range (Figure 4). TG curve of each NC sample showed closely overlapped to pristine PMMA with a sharp weight reduction observed from 350°C to 400°C (Figure 4a). The endothermic peaks at about 384.7°C in control referred to dissociation of polymeric materials. As compared to control, DSC curves for each NC also expressed similar aspects at heating range from 30°C to 600°C and DSC peaks of three NC indicated that the melting point have no significant variations (Figure 4b).
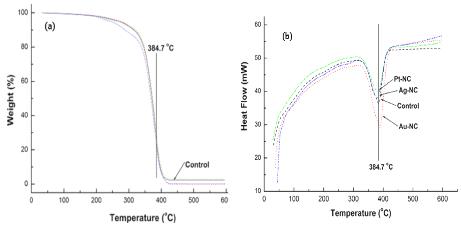
A comparative TG/DSC curves of control and NC groups loading 200 ppm of NPs under nitrogen at 10°C /min. TG curves of NC samples showed closely similar aspect to that of control (Fig. 4a) with a sharp weight reduction observed from 350°C to 400°C. DSC curves of each NC samples (Fig. 4b) expressed similar thermogram from 30°C to 600°C with the endothermic peak around 384.7°C along with control.
Color stability
The mean values of observed color differences after the addition of each NPs are shown in Table 2, when compared with control (0 ppm), the values of ΔE* were detected from 6.04 ~ 22.56 in Au-NC, 2,17 ~ 16.43 in Pt-NC and 5.48 ~ 27.18 in Ag-NC. The more dose of NPs incorporated, the significantly greater ΔE* were expressed (p < 0.01), except Au-NC between 100 and 200 ppm combined (p > 0.01). The highest color change occurred at Ag-NC containing 200 ppm combined (ΔE* = 27.18 ± 2.05) and the lowest color change was observed in Pt-NC containing 10 ppm (ΔE* = 2.17 ± 0.12). Pt-NC showed statistically lesser ΔE* than Au-NC and Ag-NC in every doses of NPs from the baseline of control (p < 0.01).
DISCUSSION
In this study, each NPs solution was synthesized by chemical reduction method which is one of the most employed and inexpensive methods to synthesize nano-sized metal. PVP was used as stabilizer to control particles growth and prevent their aggregation (Gorup et al. 2011; Monteiro et al. 2012). Although no golden standard for the assessment on antimicrobial denture materials has been established so far, FACS in this study has advantage over standard CFU (colony forming unit) or zone of inhibition growth which offer no informations about the presence of viable cells (Rodriguez & Thornton, 2008). Complemented microscopic evaluation of stained samples can detect both viable and killed cells, thus it can be used for susceptibility testing of biofilms as for determining the effectiveness of antimicrobial compounds used in oral cavity.
According to the results of FACS analysis as shown in Table 1, the antifungal character of each NC was identified as not fungicidal activity but as the significant anti-adherent effect of viable cell to that of control and there was no comparative predominance in antifungal activity among the NC group of 200 ppm dose. In 100 ppm dose group, though Ag-NC solely exhibited significant anti-adhesion effect to that of control, there were no statistical differences with Au-NC and Pt-NC. The antimicrobial mechanism of biomaterials such as NC in present study has not been fully elucidated yet. Some authors suggested nano-silver composites that release Ag in the oxidised form to medium showed strong antibacterial activity (Kassaee et al.. 2008; Kong & Jang, 2008) and composites containing NPs could act as reservoirs of metal ions to release (Hetrick & Schoenfisch, 2006). However, ion eluted could be extremely restricted due to the structure of the hydrophobic polymer network whose water uptake may not be sufficient for ion release from NPs embedded in the PMMA bulk (Nam et al., 2012; Monteiro et al., 2012). In addition, Vertex® in present study contains a cross-linking agent, ethylene glycol dimethacryl, probably ionic elution could be strongly trapped into the crosslinked polymer structure. Other reported that the biocidal effect of polymers containing nano-silver was probably due to the direct contact between the microbes and NPs (Ahn et al., 2009). However, based on FACS results, the anti-adherent effect of NC could be assumed as the “non-contact” interaction between NPs and fungi caused by an altered physicochemical interactions or a modified polarity of NC surface. Electrostatic interaction is known one of the factors that could influence the adherence of Candida to polymers (Park et al., 2003; Puri et al., 2008). Some authors suggested bacterial adhesion could be reduced on the negatively charged PMMA/ AA (acrylic acid), while increased on the positively charged PMMA/DMAEMA (dimethylamino ethyl methacrylate) (Kiremitci-Gumustederelioglou & Pesmen, 1996) or hindered if the surface chemistry of polymer is changed or modified with silver (Woodyard et al., 1996; Silver, 2003). Thus, present study implies NPs could promote pristine PMMA to be negatively charged against the negatively charged fungal cell wall which induce the dominant repulsive force (anti-adherent effect) rather than attractive force (sterilizing effect) (Campoccia et al., 2013). Regarding the toxicity to oral epithelial tissues, antimicrobial composites would rather be characterized by anti-adherent effect whose primary scope is the prevention not treatment that might cause undesirable interactions with host tissues. However, further investigations should be still required because the present study has the limitation since only one strain of C. albicans and one heat-polymerized denture base were used. To clarify the fungi-nanocomposite interaction including other unknown factors such as material hydrophobicity, surface free energy and contact angle that may also influence the adhesion need to be investigated.
Thermal analysis is general term used to describe and monitor the change of material proper during the heating process and it has a significant implication as far as polymer fabrication processes are concerned (Davy et al., 1997). In this analysis, additions of NPs did not affect the glass transition phase of the pristine polymers, TG thermograms for three NC groups were closely in accordance with control even at the highest dose, 200 ppm (Figure 4a). DSC was employed to measure the heat effects of phase transitions of NC and generally used in the research of the polymerization or setting reactions of dental resins. DSC provides a fast method for determining the glass transition temperature which is critical in understanding the upper use of the temperature and processing environs (Aouachria & Bensemra, 2006). As compared to control, NC samples exhibited a similar pattern of degradation with the endothermic peak at 384.7°C (Figure 4b), suggesting each Au, Pt and Ag nanoparticle is uniformly immobilized into PMMA resin.
Color stability is an important clinical behaviour for denture base resin since it may provide critical information on the serviceability of this materials. Despite several studies determinated the efficacy of various antimicrobial composites (Ahn et al., 2009; Wady et al., 2012; Monteiro et al., 2012), there have not been any informations in the literature regarding the color stability of polymer influenced by NPs. Generally, if ΔE* is less than 1.0, chromatic value is deemed to be very slight and clinically acceptable range is at between 1.0 and 2.0 (Johnston & Kao, 1989). However, if values were significantly greater than 2.69 which has been considered the perceptibility threshold (Chang et al., 2009), it is no longer within the limits of clinical acceptability. In this experiment, ΔE* of all NC samples found to be clinically unacceptable values except 10 ppm PtNPs incorporated (ΔE* = 2.17) after 120 hours of their curing. Pt-NC revealed significantly lesser color change as compared to Au-NC and Ag-NC and that could be an advantage on selection of noble NPs as nano-ingredient. Amongst the factors, the oxidative reaction of NPs may contribute to the discoloration of NC and although addition of NPs has antimicrobial advantage, it should be concern for its affects on the cosmetics of PMMA resin. Further studies about the different blending methods or dispersion of NPs in the monomer would be considered to solve the color problem of NC.
Conclusion
Within the limitations of this study, three noble metal nanoparticles (Au, Pt and Ag) were stably immobilized in PMMA denture acrylic respectively. These nanocomposites could be relevant in preventing fungal colonization on denture polymer surface. Addition of those nanoparticles to PMMA resulted in successful polymerization and stable physical properties through thermal analysis. However, the characteristic such as color change remains to be evaluated before recommended in the clinical use.
Notes
** The present research has been conducted by the Bisa Research Grant of Keimyung University in 2012
References
-
T Ahmad, IA Wani, N Manzoor, J Ahmed, AM Asiri, Biosynthesis, structural characterization and antimicrobial activity of gold and silver nanoparticles, Colloids Surf B Biointerfaces, (2013), 107, p227-234.
[https://doi.org/10.1016/j.colsurfb.2013.02.004]
-
SJ Ahn, SJ Lee, JK Kook, BS Lim, Experimental antimicrobial orthodontic adhesives using nanofillers and silver nanoparticles, Dent Mater, (2009), 25, p206-213.
[https://doi.org/10.1016/j.dental.2008.06.002]
-
K Aouachria, NB Bensemra, Miscibility of PVC/ PMMA blends by vicat softening temperature, viscometry, DSC and FTIR analysis, Polym Test, (2006), 25, p1010-1108.
[https://doi.org/10.1016/j.polymertesting.2006.07.007]
-
P Boomi, HG Prabu, J Mathiyarasu, Synthesis and characterization of polyaniline/Ag-Pt nanocomposite for improved antibacterial activity, J Colloids Surf B Biointerfaces, (2013), 103, p9-14.
[https://doi.org/10.1016/j.colsurfb.2012.10.044]
-
L Braydich-Stolle, S Hussain, JJ Schlager, MC Hofmann, In vitro cytotoxicity of nanoparticles in mammalian germline stem cells, Toxicol Sci, (2005), 88, p412-419.
[https://doi.org/10.1093/toxsci/kfi256]
-
D Campoccia, L Montanaro, CR Arciola, A review of the biomaterials technologies for infection-resistant surfaces, Biomaterials, (2013), 34, p8533-8554.
[https://doi.org/10.1016/j.biomaterials.2013.07.089]
-
J Chandra, PK Mukherjee, SD Leidich, FF Faddoul, LL Hoyer, LJ LL, MA Ghannoum, Antifungal resistance of candidal biofilms formed on denture acrylic in vitro, J Dent Res, (2001), 80, p903-908.
[https://doi.org/10.1177/00220345010800031101]
-
J Chang, JD Da Silva, M Sakai, J Kristiansen, S IshikawaNagai, The optical effect of composite luting cement on all ceramic crowns, J Dent, (2009), 37, p937-943.
[https://doi.org/10.1016/j.jdent.2009.07.009]
-
A Chwalibog, E Sawosz, A Hotowy, J Szeliga, S Mitura, K Mitura, M Grodzik, P Orlowski, A Sokolowska, Visualization of interaction between inorganic nanoparticles and bacteria or fungi, Int J Nanomedicine, (2010), 5, p1085-1094.
[https://doi.org/10.2147/IJN.S13532]
- IJ Davies, RD Rawlings, Mechanical properties in compression of CVI-densi®ed porous carbon/carbon composite, Compos Sci Technol, (1999), 59, p97-104.
-
KW Davy, MR Anseau, C Berry, Iodinate methacrylate copolymers as X-ray opaque denture base acrylics, J Dent, (1997), 25, p499-505.
[https://doi.org/10.1016/S0300-5712(96)00064-4]
-
LF Gorup, E Longo, ER Leite, ER Camargo, Moderating effect of ammonia on particle growth and stability of quasi-monodisperse silver nanoparticles synthesized by the Turkevich method, J Colloid Interface Sci, (2011), 360, p355-358.
[https://doi.org/10.1016/j.jcis.2011.04.099]
- EM Hetrick, MH Schoenfisch, Reducing implantrelated infections: active release strategies, Chem Soc Rev, (2006), 35, p780-789.
-
WM Johnston, EC Kao, Assessment of appearance match by visual observation and clinical colorimetry, J Dent Res, (1989), 68, p819-822.
[https://doi.org/10.1177/00220345890680051301]
-
MZ Kassaee, A Akhavan, N Sheikh, A Sodagar, Antibacterial effects of new dental acrylic resin containing silver nanoparticles, J Appl Pol Sci, (2008), 110, p1699-1703.
[https://doi.org/10.1002/app.28762]
- SA Klotz, DJ Drutz, JE Zajic, Factors governing adherence of Candida species to plastic surfaces, Infect Immun, (1985), 50, p97-101.
- M Kiremitci-Gumustederelioglu, A Pesmen, Microbial adhesion to ionogenic PHEMA, PU and PP implants, Biomaterials, (1996), 17, p443-449.
-
H Kong, J Jang, Antibacterial properties of novel poly(methyl methacrylate) nanofiber containing silver nanoparticles, Langmuir, (2008), 24, p2051-2056.
[https://doi.org/10.1021/la703085e]
-
E Lima, R E, V Lara, A Guzmán, Gold nanoparticles as efficient antimicrobial agents for Escherichia coli and Salmonella typhi, Chem Cent J, (2013), 7, p11.
[https://doi.org/10.1186/1752-153X-7-11]
- TE Motaung, AS Luyt, F Bondioli, M Messori, ML Saladino, A Spinella, G Nasillo, E Caponetti, PMMA–titania nanocomposites: Properties and thermal degradation behaviour, Polym Degrad Stabil, (2012), 97, p1325-1333.
-
DR Monteiro, LF Gorup, AS Takamiya, ER de Camargo, AC Filho, DB Barbosa, Silver distribution and release from an antimicrobial denture base resin containing silver colloidal nanoparticles, J Prosthodont, (2012), 21, p7-15.
[https://doi.org/10.1111/j.1532-849X.2011.00772.x]
-
KY Nam, CH Lee, CJ Lee, Antifungal and physical characteristics of modified denture base acrylic incorporated with silver nanoparticles, Gerodontology, (2012), 29, pe413-419.
[https://doi.org/10.1111/j.1741-2358.2011.00489.x]
- WJ O'Brien, CL Groh, KM Boenke, A new, small color difference equation for dental shade, J Dent Res, (1990), 69, p1762-1764.
- A Panácek, M Kolár, R Vecerová, R Prucek, J Soukupová, V Krystof, P Hamal, R Zboril, L Kvítek, Antifungal activity of silver nanoparticles against Candida spp, Biomaterials, (2009), 30, p6333-6340.
-
MV Park, AM Neigh, JP Vermeulen, LJ de la Fonteyne, HW Verharen, JJ Briedé, H van Loveren, WH de Jong, The effect of particle size on the cytotoxicity, inflammation, developmental toxicity and genotoxicity of silver nanoparticles, Biomaterials, (2011), 32, p9810-9817.
[https://doi.org/10.1016/j.biomaterials.2011.08.085]
- SE Park, AR Periathamby, JC Loza, Effect of surface-charged poly(methylmethacrylate) on the adhesion of Candida albicans, J Prosthodont, (2003), 12, p249-254.
- GL Polyzois, SA Yannikakis, AJ Zissis, PP Demetriou, Color changes of denture base materials after disinfection and sterilization immersion, Int J Prosthodont, (1997), 10, p83-89.
-
G Puri, DW Berzins, VB Dhuru, PA Raj, SK Rambhia, G Dhir, AR Dentino, Effect of phosphate group addition on the properties of denture base resins, J Prosthet Dent, (2008), 100, p302-308.
[https://doi.org/10.1016/S0022-3913(08)60210-3]
-
SB Rodriguez, RJ Thornton, Use of flow cytometry with fluorescent antibodies in real-time monitoring of simultaneously inoculated alcoholic-malolactic fermentation of Chardonnay, Lett Appl Microbiol, (2008), 46, p38-42.
[https://doi.org/10.1111/j.1472-765X.2007.02265.x]
-
T Saito, T Takatsuka, T Kato, K Ishihara, K Okuda, Adherence of oral streptococci to an immobilized antimicrobial agent, Arch Oral Biol, (1997), 42, p539-545.
[https://doi.org/10.1016/S0003-9969(97)00054-X]
- E Sawosz, A Chwalibog, J Szeliga, F Sawosz, M Grodzik, M Rupiewicz, T Niemiec, KA Kacprzyk, Visualization of gold and platinum nanoparticles interacting with Salmonella enteritidis and Listeria monocytogenes, Int J Nanomedicine, (2010), 5, p631-637.
- S Silver, Bacterial silver resistance: molecular biology and uses and misuses of silver compounds, FEMS Microbiol Rev, (2003), 27, p341-353.
-
A Sodagar, MZ Kassaee, A Akhavan, N Javadi, S Arab, MJ Kharazifard, Effect of silver nano particles on flexural strength of acrylic resins, J Prosthodont Res, (2012), 56, p120-124.
[https://doi.org/10.1016/j.jpor.2011.06.002]
-
I Sondi, B Salopek-Sondi, Silver nanoparticles as antimicrobial agent: a case study on E. coli as a model for Gram-negative bacteria, J Colloid Interface Sci, (2004), 275, p177-182.
[https://doi.org/10.1016/j.jcis.2004.02.012]
-
A Vanden Abbeele, H de Meel, M Ahariz, JP Perraudin, I Beyer, P Courtois, Denture contamination by yeasts in the elderly, Gerodontology, (2008), 25, p222-228.
[https://doi.org/10.1111/j.1741-2358.2007.00247.x]
-
AF Wady, AL Machado, V Zucolotto, CA Zamperini, E Berni, CE Vergani, Evaluation of Candida albicans adhesion and biofilm formation on a denture base acrylic resin containing silver nanoparticles, J Appl Microbiol, (2012), 112, p1163-172.
[https://doi.org/10.1111/j.1365-2672.2012.05293.x]
-
LL Woodyard, TL Bowersock, JJ Turek, GP McCabe, J DeFord, A comparison of the effects of several silver-treated intravenous catheters on the survival of staphylococci in suspension and their adhesion to the catheter surface, J Contr Rel, (1996), 40, p23-30.
[https://doi.org/10.1016/0168-3659(95)00135-2]
-
MS Yildirim, U Hasanreisoglu, N Hasirci, N Sultan, Adherence of Candida albicans to glow-discharge modifies acrylic denture base polymers, J Oral Rehabil, (2005), 32, p518-525.
[https://doi.org/10.1111/j.1365-2842.2005.01454.x]