
Hyaluronic Acid Immobilized Polycaprolactone 3D Scaffolds by Plasma Polymerization for MC3T3-E1 Cell Attachment and Proliferation
폴리카프로락톤 스캐폴드는 골조직공학 분야에서 널리 사용되고 있지만, 비활성의 고분자 표면 성질로 인해 생체적합성은 부족하다. 히알루론산은 세포 접착과 이동 그리고 증식에 중요한 역할을 한다. 본 연구에서는 아민 플라즈마 중합으로 폴리카프로락톤 3차원 스캐폴드에 히알루론산을 고정화하여 조골모세포의 부착과 증식에 대한 평가를 수행하였다. 표면 아민기 정량을 통하여 결정된 100W의 전력과 15 mim의 시간의 플라즈마 최적 조건에서 중합된 아민기 플라즈마 고분자박막을 폴리카프로락톤 3차원 스캐폴드에 적용하였다. 그리고 표면 아민기와 히알루론산을 공유결합하여 보다 세포 거동에 유리한 표면을 구현하였다. 물 접촉각 결과는 친수성이 증가하였음을 보여주었고, 적외선 및 광전자 분광기 분석 결과로부터 표면 개질 후 아민기와 히알루론산이 폴리카프로락톤 스캐폴드 표면에 도입되었음을 확인하였다. 이와 같이 제조한 3차원 폴리카프로락톤 스캐폴드에 대한 세포 부착과 증식 반응 평가 결과에서 표면 개질된 폴리카프로락톤 스캐폴드 표면은 세포 부착능이 증가하였으며, 향상된 수준의MTT 값을 나타내었다. 히알루론산이 고정화된 폴리카프로락톤 3차원 스캐폴드는 골조직분야에서 유망한 하나의 재료로 적용될 수 있다고 사료된다.
Keywords:
Polycaprolactone, Plasma polymerization, Allylamine, Hyaluronic acid, Tissue engineeringINTRODUCTION
The ideal scaffold for tissue regeneration should have biocompatibility, biodegradability, suitable biodegradability, easy fabrication and sufficient mechanical properties (Dzenis, 2004). In tissue engineering, the scaffold serves as a three-dimensional (3D) template for cell adhesion, proliferation and differentiation and provides an appropriate environment for the newly formed tissue (Theron et al., 2004). Open porous biodegradable polymeric scaffolds have been widely used for regenerating tissues and organs (Van Tienen et al., 2002; Yang et al., 2001; Zeltinger et al., 2001).
The synthetic, biodegradable polycaprolactone (PCL) has received considerable attention for tissue engineering, especially for bone and cartilage regeneration since it has appropriate mechanical properties, is less expensive and is easily fabricated into complicated shapes with appropriate porosity (Liu et al., 2009; Raymond and Montgomery, 2002; Yodem et al., 2008). However, one of the critical defects of the PCL-3D scaffold is the highly hydrophobic surface of the PCL, which can cause lack of surface initial cell attachment and proliferation. To improve the hydrophilicity and biological properties of PCL-3D scaffolds, various techniques have been applied (Altankov et al., 1996; Groth and Altankov, 1996; Chen et al., 2003).
Plasma is a high energy state of matter, in which a gas is partially ionized into charged particles, electrons, and neutral molecules (Dinklage et al., 2005). Plasma can provide modification of a polymer surface without using solvents or generating chemical waste and with less degradation and roughening of the material than many wet chemical treatments (Chan et al., 1996; Lane and Hourston, 1993; Ozdemir et al., 1999). The type of functionalization imparted can be varied by selection of plasma gas (Ar, N2, O2, H2O, CO2, NH2) and operating parameters (pressure, power, time, gas flow rate) (Lane et al., 1993). Plasma polymerization that has proven to be a highly successful means of developing functional interfaces for the immobilization of biomolecules and drives adhesion, spreading and proliferation of cells (Sukigaraa et al., 2004; Gu et al., 2005).
The use of a non-saturated monomer like allylamine (AAm) is advantageous because a higher amount of primary amine can be retained in the plasma polymer (Fally et al., 1995). Plasma polymerization of AAm was already used for a stable coating to change surface properties of polymeric and non-polymeric materials, to improve their wettability and incorporate amine functionalities (Kim and Kim, 2001; Toufik et al., 2002; Kuhn et al., 2002). Extremely thin plasma polymer coatings are advantageous for such strongly fixed adhesion promoting layers (Barbara et al., 2007). The AAm was chosen as the precursor for film deposition based on the good retention of amino groups (Kuhn et al., 2001).
Hyaluronic acid (HA) is a naturally occurring, biocompatible, and biodegradable polysaccharide composed of repeating disaccharide units of D-glucuronic acid and N-acetyl-D-glucosamine linked by alternating β (1 →4) and β (1→3) linkages (Ding et al., 2002). Furthermore, HA plays an important role in adhesion, migration and proliferation (Wang et al., 2009). HA is negatively charged due to the carboxyl group of glucuronic acid. Therefore, a biomaterial surface with an opposite charge can boost the first contact of the cell to the surface until the cell synthesizes matrix proteins to mediate the fundamental integrin contact in focal adhesion (Ding et al., 2002).
In this study, preosteoblast cell (MC3T3-E1) attachment and proliferation is evaluated on HA immobilized PCL-3D scaffolds through amine plasma polymerization. Optimization of plasma polymerization process was carried out for maximizing the amine functional group on PCL-3D scaffold surface. By improving the immobilization of HA on PCL-3D scaffolds, the enhancement of cell attachment and proliferation was investigated.
MATERIALS AND METHODS
1. Materials
PCL-3D scaffolds (3D Insert PCL, 3D Biotek, New Jersey, USA) were used as substrate. Polycaprolactone pellets with average molecular weight of 80,000 (Sigma-Aldrich CHEMIE GmbH, MO, USA), allylamine (Sigma-Aldrich CHEMIE GmbH, MO, USA), EDC (1-ethyl-3-[3dimethylaminopropyl] carbodiimide hydrochloride (Sigma-Aldrich CHEMIE GmbH, MO, USA), NHS (Nhydroxysuccinimide, Sigma-Aldrich CHEMIE GmbH, MO, USA), sodium dihydrogen phosphate and potassium carbonate were purchased from Aldrich. Methyl orange (MO) and hyaluronic acid (HA, TOKYO CHEMICAL INDUSTRY Co., LTD. TOKYO, JAPAN) was purchased from Tokyo Chemical Industry. All other chemicals and bioreagents were used without further purification. The density of amine groups on the PCL films (PCL-F) was quantitatively determined by using MO test. The PCL-F was prepared by dissolving 12.5 g of PCL pellets in 50 mL of chloroform. The polymer solution was then cast onto a glass substrate and the solvent was removed by controlled evaporation at room temperature over a period of 24 h. The obtained pristine PCL-F with a thickness of 40-100 was washed with copious amounts of an alcohol/water (1/1, v/v) mixture. The dried PCL-F was cut into specimen size of 20 mm (diameter) in order to use the samples in this experiment.
2. Plasma polymerization
The surface modification of the PCL-3D scaffolds was carried out by depositing an ultra-thin polymeric layer containing amine groups through plasma polymerization of AAm using radio frequency (RF) discharge device (model UM1000134-001A mini plasma station, PLASMART, Korea). The system consists of three main parts: (i) a reaction chamber, (ii) a RF generator, and (iii) a vacuum system. The pressure was decreased to 10-3 torr using a vacuum pump and the plasma was generated with a RF power generator. The monomer vapors were supplied to the reactor chamber from liquid allylamine contained in cylindrical flask via a stainless steel line heated at 30°C (20 sccm). Plasma polymerization was carried out at a various discharge power (30, 50, 70, and 100 W) for various deposition times (5, 10, and 15 min). PCL -F and PCL-3D deposited with plasma polymerized AAm thin film are referred to as “PCL-F/NH2” and “PCL-3D/NH2”.
3. HA immobilization
A natural polymer HA was immobilized onto the – NH2 functionalized surface in an EDC-NHS aqueous solution at a concentration of 2.5 mg/mL (EDC/NHS = 4/1 (w/w)) for 2 h with gentle shaking at 0-4 °C (Shi et al., 2007). The samples were then immersed in a 0.3 g/L HA solution for 24 h with mild stirring at 0-4 °C. The primary amine (H2N-) of the PCL-3D/NH2 surface was reacted with the COOH group of the HA molecules. The prepared samples were rinsed with distilled water to remove the unbound HA. The PCL-F/NH2 and PCL-3D/NH2 immobilized with HA are referred to as “PCL-F/NH2/HA”, and “PCL-3D/NH2/HA”.
4. Determination of amino groups
Methyl orange (MO) is an acid dye which binds with amino group containing substrates, and the dye can be removed again by bases (Gurr, 1971). MO colorimetric measurement can be used to determine the number of amino groups on polymers surfaces. The amino functionalized samples were treated with the acidic methyl orange solution (0.1 mM MO in a 0.1 M solution of sodium dihydrogen phosphate in water, filtered; pH app. 4.7) for 1 h. After rinsing the excess of dye with water the samples were carefully pressed between tissue papers to remove the water. The fixed methyl orange was resolved by 3 mL of a 0.1 M potassium carbonate solution and the concentration determined photometrically at 460 nm (Hartwig and Mulder, 1994). The -NH2 content was obtained from a calibration plot of the optical density versus dye concentration with a 1:1 ratio assumed between the dye and amino groups (Zuwei et al., 2007).
5. Surface characterization
After plasma treatment, the hydrophilicity of the surface was determined by contact angles using dynamic contact angle measurements (Contact-Angle, GS, Surface Tech. Co. Ltd. Korea). The chemical structure of the coated polymer matrix was analyzed by ATR-FTIR (IFS66V/S & HYPERION 3000, Bruker Optiks, Germany). The surface chemical compositions of the samples were analyzed in X-ray photoelectron spectroscopy (XPS, Multilab 2000 system, SSK, USA). The change of the surface morphology was observed by field emission scanning electron microscopy (FE-SEM, Hitachi, S-4800, Japan).
6. MC3T3-E1 cell culture
MC3T3-E1 (ATCC CRL-2953) cell, a clonal preosteoblastic cell line derived from newborn mouse calvarias, were cultured in α-MEM medium and 5% CO2 at 37 °C with cell seeding density of 1 × 104 cell/mL.
7. Cell attachment and proliferation assay
The samples from all groups were prefixed with a mixed solution containing 2.5% glutaraldehyde and 2.5% paraformaldehyde for 3 hours, washed three times for 10 min each in the phosphate buffer, and post-fixed in 1% osmium tetroxide for 30 min. The samples were dehydrated in a graded series of aqueous ethanol solutions (70%, 90%, 95%, and 100%) for 5 min each. The samples were then placed in hexamethyl-di-silazane (Fluka) to remove any alcohol. After 10 min, the samples were removed and allowed to air dry overnight at room temperature. The sample was coated with a thin layer of gold using an automated sputter for 1 min. Representative digital images of each sample were observed using FE-SEM. The proliferation of MC3T3-E1 cells on substrates was examined by MTT (3-(4, 5-dimethylthiazol-2-yl)-2, 5-diphenyltetrazolium bromide, Sigma-Aldrich CHEMIE GmbH, MO, USA) assay after 1, 3, and 5 days of culture. All samples were placed into a 12-well plate and seeded with a density of 2 × 105 cells/mL. MTT assay was described previously (Seo et al., 2010).
8. Statistical analysis
The results of the MTT assay were analyzed statistically using a Student’s t test. The statistical significance was considered at p < 0.05.
RESULTS AND DISCUSSION
1. Analysis of surface modified PCL film
To investigate the -NH2 concentration on the plasma treated PCL-F surfaces, MO test was used. By assuming a combination ratio of 1:1 between MO and -NH2, Hartwig et al. measured the amino group density on ammonium plasma treated polymer surface, which was as low as several nano-mole per square centimeters (Hartwig and Mulder, 1994).
Figure 1 shows the effect of the time and RF input power on the surface density of the amino groups. It was found to increase as a function of the plasma treated time and RF power applied. We determined the optimum condition (100 W, 15 min) of the plasma polymerization process in Figure 1 data and then all samples was treated at optimum condition.
The contact angles of the PCL-F, PCL-F/NH2, and PCL-F/NH2/HA surface were 71.6 ± 4.6˚, 45.0 ±7.3˚, and 33.1 ±1.4˚ respectively (n=5). It is clearly shown that the chemical composition of plasma polymers influences the wettability of surfaces. Functional groups like amine- carboxyl-, or hydroxyl groups are known to have a hydrophilic character. Polymers obtained by high-energetic plasma treatment having high amine content were found to be more hydrophilic than others (Hamerli et al., 2002). The PCL-F/NH2 and PCL-F/HN2/HA surface shows low contact angle compared with PCL-F surface. Hydrophilic surface shows better cell adhesion on implant materials than hydrophobic surfaces (Foest et al., 2008; Hartwig and Mulder, 1994).
Figure 2 shows the ATR-FTIR spectra of the PCL-F, PCL-F/NH2 and PCL-F/NH2 /HA surfaces. ATR-FTIR spectrometry is a very powerful technique for detecting the inter-molecular reactions between the two polymers (Kaminśka and Sionkowska, 1996). Several characteristic bands of PCL were observed at 2949 cm-1 (asymmetric CH2 stretching), 2865 cm-1 (symmetric CH2 stretching), 1727 cm-1 (carbonyl stretching) (Wan et al., 2009; Ghasemi-Moarakeh et al., 2010). The ATR-FTIR spectrum of PCL-F/NH2 showed bands at 3380 due to N-H stretching of amide bond, N-H bending at 1543 cm-1. The broader band between 1550 and 1680 cm-1 can be assigned to the stretching modes of C=C bonds, imines, amines and amides groups (Lin et al., 2011; Meskinfam et al., 2011). After HA was immobilized onto PCL-F/NH2 samples, the band near 1500 cm-1 decreased. The weak peak near 1700 cm-1 for the PCL-F/NH2/HA sample was assigned to the carbonyl in amide group derived from the -COOH terminals of HA reacted with the -NH2 terminals of PCL-F/NH2 on the surface through covalent bonding. ATR-FTIR confirmed that HA had been immobilized successfully onto the PCL-F/NH2 surface through crosslinking via EDC.
Figure 3 and Table 1 show the surface chemical composition of PCL-3D, PCL-3D/NH2 and PCL-3D/NH2/ HA surfaces. XPS analysis was used to examine each reaction step because it can provide information on chemical bonds and atomic concentrations. Table 1 lists the change in the chemical components on each surface. The chemical component ratio (O1s/C1s, N1s/C1s) changed and atomic elemental O1s significantly increased after immobilization of the HA. PCL-3D/NH2/HA surfaces had the higher content of oxygen in its molecules. Thus, the O1s/C1s of PCL-3D/NH2/HA surface was higher than that of the other. This suggests the formation of the target molecule.
The morphology after plasma polymerization and HA immobilization were examined by FE-SEM. It was revealed that the surface morphology of PCL-3D, PCL-3D/NH2 and PCL-3D/NH2/HA was not changed significantly with each different treatment, as shown in Figure 4 (a), (b), and (c), respectively.
2. Biological evaluation of modified surfaces
To observe the initial cell adhesion on the different PCL scaffolds, MC3T3-E1 cells were cultured for one day. Figure 5 shows the morphology of cells grown on the different PCL scaffolds. Cells grown on PCL-3D/NH2/HA surfaces have a spread out aspect and show multiple microvilli on the surface and long fine cytoplasmic extensions in multiple directions. In addition, these cells demonstrate apparently greater spreading with large lamellipodes indicating an active cell migration. On the HA immobilized samples the cell showed the good cytoplasmic extensions, and the cell almost reached confluence, forming a thick cell layer.
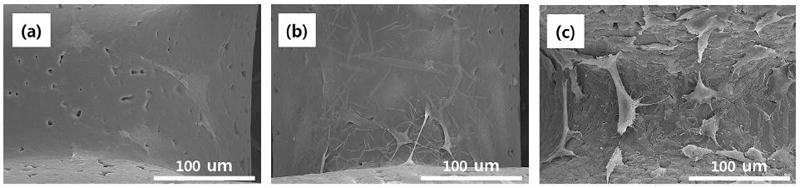
FE-SEM images of the MC3T3-E1 cells grown on the PCL 3D scaffolds for 1 day of culturing: (a) PCL-3D, (b) PCL-3D/NH2 and (c) PCL-3D/NH2/HA.
The samples used were PCL-3D, PCL-3D/NH2 and PCL-3D/NH2/HA with the PCL-3D as the control at 1 day and 5 days. The cell viability (proliferation) was assessed using a MTT assay, which is a direct mitochondrial activity assay. Figure 6 shows the viability of MC3T3-E1 seeded on the prepared samples at 1, 3, and 5 days. The cell viability is expressed as a percentage of the control value. The initial adhesion (1 day after cell seeding) of the amino group functionalized sample (PCL-3D/NH2) and HA immobilized sample (PCL-3D/NH2/HA) increased to 42% and 48% compared to the PCL-3D. This indicates that –NH2 groups on the surface can have some positive effects on the implant biocompatibility. In particular, the PCL-3D/NH2/HA surface showed a significant increase in cell viability after 5 days compared to that of the PCL-3D by approximately 44%, which was attributed to the improved adhesion and spreading ability of HA. At 5 days cell proliferation was significantly higher on the PCL-3D/NH2/HA than on the PCL-3D. Some studies demonstrated that hyaluronic acid promotes cell adhesion (Arima and Iwata, 2007). The MTT results in the present study indicate that the immobilization of HA can have some positive effects on implant biocompatibility. This confirms that the enhancement of MC3T3-E1 cell proliferation requires the presence of HA. Along with the excellent behavior of the PCL-3D/NH2/HA, the cell responses showed good cell viability on the PCL-3D/NH2/HA with a significantly higher level of cell growth than those on the PCL-3D (Figure 5 and 6).
CONCLUSION
In this study, MC3T3-E1 cell attachment and proliferation of HA immobilized PCL scaffolds were fabricated by amine plasma polymerization. The degree of –NH2 grafting density and hydrophilicity was related to plasma power and plasma treated times. XPS and FT-IR spectra confirmed the occurrence of covalent attachment of HA on the surface of amine plasma treated PCL 3D scaffold. The in vitro biological assay showed that introducing HA to the PCL-3D/NH2 surfaces had a beneficial effect on cell attachment and proliferation. The HA immobilization PCL 3D scaffolds has a good potential for eventual use in bone tissue engineering and other biomedical applications. However, further studies will be needed to clarify the role of HA on the biocompatibility.
Acknowledgments
본 논문은 2013년도 조선대학교 학술연구비의 지원을 받아 연구되었음.
References
-
Y Dzenis, Spinning continuous fibers for nanotechnology, Science, (2004), 304, p1917.
[https://doi.org/10.1126/science.1099074]
-
SA Theron, E Zussman, AL Yarin, Experimental investigation of the governing parameters in the electrospinning of polymer solutions, Polymer, (2004), 465, p2017.
[https://doi.org/10.1016/j.polymer.2004.01.024]
-
TG Van Tienen, RGJC Heijkants, P Buma, JH De Groot, AJ Pennings, RPH Veth, Tissue ingroth and degradation of two biodegradable porous polymers with different porosities and pore sizes, Biomaterials, (2002), 23, p1731.
[https://doi.org/10.1016/S0142-9612(01)00280-0]
-
S Yang, KF Leong, Z Du, CK Chua, The design of scaffolds for use in tissue engineering Part 1. Traditional Factors, Tissue Eng, (2001), 7, p679.
[https://doi.org/10.1089/107632701753337645]
-
J Zeltinger, JK Sherwood, DA Graham, R Mueller, LG Griffith, Effect of pore size and void fraction on cellular adhesion, proliferation, and matrix deposition, Tissue Eng, (2001), 7, p557.
[https://doi.org/10.1089/107632701753213183]
-
FJ Liu, R Guo, MW Shen, SY Wang, XY Shi, Effect of Processing Variables on the Morphology of Electrospun Poly[(lactic acid)-co-(glycolic acid)] Nanofibers, Macromaol Mater Eng, (2009), 294, p666.
[https://doi.org/10.1002/mame.200900110]
- HM Raymond, DC Montgomery, Response Surface Methodology: process and product optimization using designed experiment, A Wiley Interscience Publication, (2002).
-
OS Yordem, M Papila, YZ Menceloglu, Effects of electrospinning parameters on polyacrylonitrile nanofiber diameter: An investigation by response surface methodology, Materials & Desig, (2008), 29, p34.
[https://doi.org/10.1016/j.matdes.2006.12.013]
- G Altankov, F Grinnell, T Groth, Studies on biocompatibility of materials: Fibroblast reorganization of substratum-bound fibronectin on surfaces varying in wettability, J Biomed Mater Res, (1996), 30, p385.
- T Groth, G Altankov, Studies on the cellbiomaterial interaction: role of tyrosine phosphorylation during fibroblasts spreading on surfaces varying in wettability, Biomaterials, (1996), 17, p1227.
-
M Chen, PO Zamora, P Som, LA Pena, S Osaki, Cell attachment and biocompatibility of polytetrafluoroethylene (PTFE) treated with glow-discharge plasma of mixed ammonia and oxygen, J Biomater Sci Polym Ed, (2003), 14, p917.
[https://doi.org/10.1163/156856203322381410]
-
A Dinklage, T Klinger, G Marx, L Schweikhard, Plasma physics: confinement, transport and collective effects, New York Springer, (2005), 3, p540.
[https://doi.org/10.1007/b103882]
-
CM Chan, TM Ko, H Hiraoka, Polymer surface modification by plasmas and photons, Surf Sci Rep, (1996), 24, p3.
[https://doi.org/10.1016/0167-5729(96)80003-3]
-
JM Lane, DJ Hourston, Surface treatments of polyolefins, Prog Org Coat, (1993), 21, p269.
[https://doi.org/10.1016/0033-0655(93)80044-B]
-
M Ozdemir, CU Yurteri, H Sadikoglu, Physical polymer surface modification methods and applications in food packaging polymers, Crit Rev Food Sci, (1999), 39, p457.
[https://doi.org/10.1080/10408699991279240]
-
S Sukigara, M Gandhi, J Ayutsede, M Micklus, F Ko, Regeneration of Bombyx mori silk by electrospinning, Polymer, (2004), 45, p3701.
[https://doi.org/10.1016/j.polymer.2004.03.059]
-
SY Gu, J Ren, GJ Vancso, Process optimization and empirical modeling for electrospum polyacrylonitrile (PAN) nanofiber precursor of carbon nanofibers, European polym J, (2005), 14, p2559.
[https://doi.org/10.1016/j.eurpolymj.2005.05.008]
-
F Fally, C Doneux, J Riga, JJ Verbist, Quantification of the functional groups present at the surface of plasma polymers deposited from propylamine, allyamine, and propargylamine, J Appl Polym Sci, (1995), 56, p597.
[https://doi.org/10.1002/app.1995.070560509]
-
HI Kim, SS Kim, Fabrication of reverse osmosis membrane via low temperature plasma polymerization, J Membr Sci, (2001), 190, p21.
[https://doi.org/10.1016/S0376-7388(01)00380-5]
-
M Toufik, A Mas, V Shkinev, A Nechaev, A Elharfi, F Schue, Improvement of performances of PET track membranes by plasma treatment, Eur Polym J, (2002), 38, p203.
[https://doi.org/10.1016/S0014-3057(01)00130-6]
- N Barbara, F Birgit, L Frank, B Claudia, S Karsten, R Joachim, L Klaus, O Andreas, Improves initial osteoblast functions on amino-functionalized titanium surface, Biomol Eng, (2007), 24, p447.
-
G Kuhn, I Retzko, A Lippitz, W Unger, J Friedrich, Homofunctionalized polymer surfaces formed by selective plasma processes, Surf Coat Technol, (2001), 142, p494.
[https://doi.org/10.1016/S0257-8972(01)01056-8]
-
B Ding., Kim H.Y., Lee S.-C., Shao C.-L., Lee D.-R, Park S.-J, Kwag G.-B, Choi K.-J, Preparation and characterization of a nanoscale poly (vinyl alcohol) fiber aggregate produced by an electrospinning method, J Polym Sci Pol Phys, (2002), 40, p1261.
[https://doi.org/10.1002/polb.10191]
-
Y Wang, L Guo, L Ren, S Yin, J Ge, Q Gao, A study on the performance of hyaluronic acid immobilized chitosan film, Biomed Mater, (2009), 4, p035009.
[https://doi.org/10.1088/1748-6041/4/3/035009]
-
Z Shi, KG Neoh, ET Kang, CH Poh, W WANG, Bacterial adhesion and osteoblast function on titanium with surface-grafted chitosan and immobilized RGD peptide, J Biomed Mater Res, (2007), 86, p865.
[https://doi.org/10.1002/jbm.a.31648]
- E Gurr, Synthetic Dyes in Biology, Medicine and Chemistry: Academic Press, (1971), 202.
-
A Hartwig, M Mulder, Surface amination of poly (acrylonitrile), Adv. Colloid Interface Sci, (1994), 52, p65.
[https://doi.org/10.1016/0001-8686(94)80040-5]
- M Zuwei, M Zhengwei, G Changyou, Surface modification and property analysis of biomedical polymers used for tissue engineering, Colloid Surf B, (2007), 60, p137.
-
P Hamerli, TH Weigel, TH Groth, D Paul, Surface properties of and cell adhesion onto allylamine-plasma-coated polyethylenterephtalat membranes, Biomaterials, (2003), 24, p3989.
[https://doi.org/10.1016/S0142-9612(03)00312-0]
-
KL Ou, YH Shih, CF Huang, CC Chen, CM Liu, Enhanced initial cell responses to chemically modified anodized titanium, Implant Dent Relat Res, (2008), 10, p55.
[https://doi.org/10.1111/j.1708-8208.2007.00061.x]
-
KL Ou, YH Shih, CF Huang, CC Chen, CM Liu, Preparation of bioactive amorphous-like titanium oxide layer on titanium by plasma oxidation treatment, Appl Surf Sci, (2008), 255, p2046.
[https://doi.org/10.1016/j.apsusc.2008.06.189]
-
A Kaminśka, A Sionkowska, The effect of UV radiation on the thermal parameters of collagen degradation, Polym Degrad Stabil, (1996), 51, p19.
[https://doi.org/10.1016/0141-3910(95)00158-1]
-
Y Wan, X Lu, S Dalai, J Zhang, Thermophysical properties of polycaprolactone/chitosan blend membranes, Thermochim Acta, (2009), 487, p33.
[https://doi.org/10.1016/j.tca.2009.01.007]
- L Ghasemi-Moarakeh, MP Prabhakaran, M Morshed, MH Nasr-Esfahani, S Ramakrishna, Biofunctionalized PCL nanofibrous scaffolds for nerve tissue engineering, Mater Sci Eng C, (2010), 30, p1129.
-
YC Lim, J Johnson, Z Fei, Y Wu, DF Farson, JJ Lannutti, HW Choi, LJ Lee, Mircopatterning and characterization of electrospun poly(ε -caprolactone)/ gelation nanofiber tissue scaffolds by femtosecond laser ablation for tissue engineering applications, Biotechnol Bioeng, (2011), 108, p116.
[https://doi.org/10.1002/bit.22914]
- M Meskinfam, MS Sadjadi, H Jazdarreh, Biomimetic preparation of nano hydroxyapatite in gelatin-starch matrix, World Acad Sci Eng Technol, (2011), 52, p395.
-
Y Arima, H Iwata, Effect of wettability and surface functional groups on protein adsorption and cell adhesion using well-defined mixed selfassembled monolayers, Biomaterials, (2007), 28, p3074.
[https://doi.org/10.1016/j.biomaterials.2007.03.013]