
공동배양에 의한 파골세포 생성에서 티타늄 나노구조의 억제 기전
Abstract
Previously, many studies investigating differentiation of osteoclasts, osteoblasts or mesenchymal stem cells alone on titanium with nanotubular structure were reported. However, there is barely information about the effects of oxidized titanium (TiO2) nanotubular structure on the formation of osteoclast under coculture condition. In this study, we investigated the differentiation and formation of osteoclast cultured on TiO2 nanotubular structure under coculture condition. Bone marrow cells were cultured with calvarial osteoblast cells on TiO2 nanotubes with different diameters (30-100 nm). The formation of mature osteoclasts was apparently increased on 30 nm TiO2 nanotubes compared to that of electropolished titanium surface (Ti), and then dramatically reduced by increasing nanotube diameters up to 100nm. However, the adhesion of osteoclasts or osteoblasts, and the expression of osteoblast differentiation marker genes were not shown any significant difference at all diameters of TiO2 nanotubular structures. Rather, the expression of osteoclast differentiation factors (macrophage colony-stimulating factor, M-CSF, osteoprotegerin, OPG and receptor activator of nuclear factor kappa-B ligand, RANKL) produced by osteoblasts was dramatically changed by diameters of TiO2 nanotubular structure. The expression of M-CSF and OPG in osteoblasts was up-regulated, and the expression of RANKL and RANKL/OPG ratio were down-regulated by increasing diameters of TiO2 nanotubes, in particular, at 70 and 100 nm. These data suggest that the inhibition of osteoclast formation by TiO2 nanotube having 70-100 nm diameters may due to diminishing function of osteoblast responsible for osteoclast differentiation under coculture condition. It also indicate that the nano-topography on the Ti surface may affect to successful medical application including dental implantation.
Keywords:
Osteoclast, Osteoblast, TiO2 nanotube, Coculture, RANKL, M-CSF, OPGⅠ. 서론
골 항상성(bone homeostasis)과 골 리모델링(bone remodeling)은 골기질(bone matrix)을 형성하는 조골세포(osteoblasts)와 골기질을 흡수하는 파골세포(osteoclasts)의 정교한 조절을 통하여 유지되며, 이러한 세포들에 의한 골대사(bone metabolism) 조절의 불균형으로 인하여 골다공증, 류마티스 관절염, 치주질환 등의 골연관(bone-related) 질환이 유도된다(Walsh 등, 2006). 단핵세포/대식세포(monocyte/macrophage)로부터 파골세포로의 분화는 macrophage colony-stimulating factor (M-CSF)와 receptor activator of nuclear factor–κB ligand (RANKL)에 의해 유도될 수 있다. RNAKL이 파골세포 전구세포 표면에 있는 수용체인 receptor activator of nuclear factor–κB (RANK)에 결합하면 다양한 단백질들에 의한 세포 내 신호전달 과정을 거쳐 nuclear factor of activated T cells, cytoplasmic 1 (NFATc1) 같은 전사인자의 발현을 증가시켜 파골세포로의 분화와 생성을 유도한다(Takayanagi 2007; Kim and Kim 2014). RANKL은 1α,25-(OH)2D3 (VitD3), interleukin-1 (IL-1), and 부갑상선 호르몬(parathyroid hormone, PTH) 등과 같은 다양한 인자들의 자극에 의해 조골세포에 의해 생성된다(Huang 등. 2004). 또한 이러한 다양한 인자들의 자극에 의해 조골세포는 RANKL에 대한 미끼 수용체(decoy receptor)로 알려진 osteoprotegerin (OPG)을 분비하여 파골세포의 분화와 생성을 조절한다(Hofbauer 등. 1998; Hofbauer 등. 1999). 이러한 RANKL/RANK/OPG axis의 정교한 조절과 균형은 골 대사 및 골 항상성 뿐 아니라 면역시스템에서도 중요한 역할을 한다(Walsh and Choi 2014).
티타늄과 그 합금은 고품질의 기계적 특성, 부식에 대한 내성 및 생체 적합성으로 인해 오랫동안 이식 가능한 생체 적합 물질로 사용되어 왔다(Albrektsson와 Wennerberg, 2005; Le Gu´ehennec 등, 2007). 이전의 연구에서, 티타늄 (Ti)은 면역 반응이나 결합 조직층을 형성하는 foreign body 반응 없이 뼈 표면과 우수한 물리적 결합을 제공할 수 있음이 밝혀졌다(Linder et al., 1988; Satomi et al., 1988). 최근, 나노구조는 마이크로구조보다 높은 표면적/부피 비율과 훨씬 우수한 가소성을 보인다고 알려졌다(Mor et al., 2006). 또한, TiO2 나노 튜브의 생물학적 응용 측면에서 TiO2 나노 튜브는 조골 세포의 접착, 증식 및 기능을 촉진함이 밝혀졌고(Oh 등, 2006: Brammer 등, 2009), 연골세포의 기능적 특성과 내피세포(endothelial cell)의 이동성을 향상시킨다고 알려졌다(Brammer et al., 2008). 또한, TiO2 나노 튜브 표면에서 파골세포의 부착과 증식이 증가되었다(Park et al., 2009). 최근 몇 년 동안, 나노 스케일의 표면 변형은 나노 스케일의 지형이 세포 부착과 조골세포 및 파골세포 분화에 영향을 미친다는 연구 결과가 발표된 이후로 더욱 주목을 받아왔다(Bertoncini 등, 2012; Nagasawa 등, 2015). 또한 TiO2 나노 튜브 표면에서의 세포 습성(behavior)은 나노튜브 직경에 따라 변함이 보고되었다. 특히 Oh 등은 70 nm TiO2 나노튜브에서 단백질, 조골세포 및 중간엽줄기세포(MSC)의 접착력이 가장 높았고, 조골세포 및 중간엽줄기세포의 신장 및 세포 활성도는 70-100 nm 나노튜브에서 가장 높게 나타났다고 보고하였다(Oh 등, 2006; Brammer 등, 2009). 한편, Park 등은 15 nm 나노튜브가 조골세포, 중간엽줄기세포 및 파골세포의 증식과 분화에 최적이라고 보고하였다(Park 등, 2009; Park 등, 2007). 그러나 다양한 직경을 가지는 TiO2 나노튜브 위에 생체 내 조건과 유사하게 골수세포와 조골세포를 동시에 배양하면서 파골세포의 생성과 그 조절 기전을 연구한 보고는 현재 거의 없다.
따라서 본 연구의 목적은 다양한 직경을 가지는 TiO2 나노튜브 위에서 공동배양을 통한 파골세포 생성 및 조절기전을 규명하여 최적의 직경을 가지는 TiO2 나노튜브의 임상적 적용에 대한 타당성을 제공하는 것이다.
Ⅱ. 재료 및 방법
1. 사용 동물 및 시약
본 연구에 사용된 모든 생쥐는 (주)오리엔트 바이오(성남, 대한민국)에서 6-8 주령의 C57BL/6를 구입하였고, 모든 동물실험은 원광대학교 동물실험윤리위원회의 승인(WKU16-5)을 받아 시행하였다. 파골세포 분화에 필요한 사이토카인인 RANKL과 M-CSF는 김태수 박사(한국한의학연구원, 대전, 대한민국)로부터 기증받았다.
2. 티타니아 (TiO2) 나노튜브 시편 제작
아세톤, 에탄올, 및 증류수의 순서로 세척한 티타늄 판(두께: 200 μm, 순도: 99.5%, 현대 티타늄, 대한민국)을 6 wt% Perchloric acid (Sigma, USA), 37 wt% Butoxy ethylene glycol (Junsei, Japan), 59 wt% 메탄올 (Sigma, USA)을 이용하여 영하 40℃ 에서 30분 동안 전해연마(electropolished) 시켰다(Oh 등, 2015). 전해연마된 티타늄 판을 0.5 wt% 불산(순도: 48%, EM science, USA)와 아세트산(순도: 98%, Fisher, USA)을 전해질 용액으로 하여 5-20 V의 범위에서 30분 동안 양극산화시켜 30-100 nm 직경의 TiO2 나노튜브층을 합성하였다. 양극산화 후 시편을 증류슈로 세척하고 80℃ 오븐에서 24시간 건조한 후, 500℃에서 2시간 동안 열처리를 하였다(공기 중 열처리, 승온 및 냉각속도 = 1 K/min). 열처리된 TiO2 나노튜브 시편은 1.27⨯1.27 cm2로 절단하고, 증류수에 시편을 넣은 후, 증류수와 함께 고압증기 멸균시킨 후 실험에 사용하였다.
3. 공동배양(Coculture)을 통한 파골세포 생성 및 확인
공동배양을 통한 파골세포 생성을 위해, 생쥐의 대퇴골(femur)과 경골(tibia)에서 분리한 골수세포(bone marrow cells, BMs)와 두개골(calvaria)에서 분리한 조골세포(osteoblasts)를 사용하였다(Lee 등, 2008). 10% fetal bovine serum (FBS, HyClone, USA)과 5⨯10-8M 1,25-dihydroxyvitamin D3 (VitD3, Sigma, Korea) 첨가된 Minimum Essential Medium Alpha (MEM-α, HyClone, USA) 배지가 들어 있는 24 well plate에 TiO2 나노튜브 시편을 넣은 후 골수세포(6⨯105cells/well)와 조골세포(6⨯104cells/well)를 plate에 같이 넣고 37℃ CO2 배양기에서 9일 동안 배양하였다. 배양 후 TiO2 나노튜브 시편에 생성된 성체 파골세포(mature osteoclast)를 확인하기 위하여 0.5% Trypsin-EDTA를 배양된 세포에 1분 동안 처리하여 조골세포를 제거한 후 2.5% fluorescein diacetate (FDA, Sigma, Korea)를 처리하여 파골세포를 염색하였다. 100 nm 이상의 직경을 가진 성체 다핵파골세포(multinucleated osteoclasts, MNCs) 숫자를 도립형광현미경(DM IL LED, Leica, Korea)으로 측정하였다. 생성된 단핵, 이핵 또는 다핵파골세포의 tartrate-resistant acidic phosphatase (TRAP) 효소 활성을 측정하기 위하여 TiO2 나노튜브 시편에 생성된 파골세포를 10% 포말린(formalin)으로 고정하고 TRAP 기질 용액(1 mg/ml ρ-NPP dissolved in TRAP buffer, pH 5.2)을 첨가하였다(Lee 등, 2008). 30분 후 반응액을 새 ELISA plate로 옮긴 후 1N 수산화나트륨(NaOH)을 넣어 반응을 종결하였다. ELISA 기기를 이용하여 405 nm에서 흡광도를 측정하였다.
4. 세포 부착능
파골세포의 TiO2 나노튜브 시편에서의 부착능을 측정하기 위하여 생쥐에서 분리한 골수세포를 30 ng/ml의 M-CSF 처리 하에 3일 동안 배양하여 파골세포 전구세포인 골수유래대식세포(bone marrow-derived macrophages, BMMs)를 만들었다. BMMs (6⨯104cells)을 TiO2 나노튜브 시편 위에 넣고 10% FBS, M-CSF (50 ng/ml)가 첨가된 α-MEM 배지에서 2일 동안 배양하였다. 두개골에서 분리한 조골세포의 부착능을 측정하기 위하여 조골세포(6⨯104cells)를 TiO2 나노튜브 시편 위에 넣고 10% FBS, 5⨯10-8 M VitD3가 첨가된 α-MEM 배지에서 3일 동안 배양하였다. 배양 후 PBS로 세척하고 2.5% FDS로 부착된 세포를 염색하였다. TiO2 나노튜브 시편 중 임의의 6개의 영역에 부착된 세포의 수를 측정하였다.
5. 역전사 중합효소연쇄 반응(RT-PCR)을 통한 조골세포 분화 및 기능 확인
조골세포의 분화를 확인하기 위하여, 두개골 유래 조골세포를 TiO2 나노튜브 시편 위에 놓고 10% FBS를 함유하는 α- MEM 배지에서 9일 동안 L-ascorbicacid (50 ug/ml), 및 β- glycerophosphate (10 mM)을 처리하여 배양하며 조골세포 분화를 유도하였다. 한편, 파골세포 분화를 유도하는 조골세포의 기능을 측정하기 위하여, 조골세포를 TiO2 나노튜브 시편 위에 놓고 10% FBS를 함유하는 α-MEM 배지에서 7일 동안 VitD3 (5⨯10-8 M)을 처리하여 파골세포 분화유도 인자들의 발현을 유도하였다. 이와 같이 배양한 조골세포에 TRIzol reagent (Invitrogen, USA)를 처리하여 RNA를 추출한 다음 20 μl 반응액에 1μg의 RNA 및 random primers 넣고 SuperScript II RT-PCR System (Invitrogen)을 사용하여 42℃에서 50분 동안 반응시켜 cDNA를 합성하였다. 그 다음, 조골세포 분화인자 유전자(Collagen type1 alpha 1 (Col1α1), osteocalcin (OCN), osteopontin (OPN)) 및 파골세포 분화유도 인자 유전자(M-CSF, RANKL, OPG)들의 발현을 PCR을 통하여 확인하였다. 이 실험에 사용된 primer는 Cosmo Genetech Inc. (Seoul, Korea)에서 합성하였고, 그 서열은 Table 1에 표시하였다.
6. 통계분석
정량분석에 관한 모든 실험 결과는 Student’s t-test를 사용하여 분석되었고, 평균±표준편차(SD)로 표현되었다. P ≤ 0.05의 경우에만 통계학적 유의성이 있다고 판단하였다. 모든 실험은 반복되었고 대표 결과만 표시하였다.
Ⅲ. 결과
1. 전해연마된 티타늄(Ti) 표면에 다양한 직경을 가진 나노튜브 제조
5-20 V의 양극산화 전압의 변화를 통하여 30-100 nm 직경을 가진 나노튜브를 전해연마된 티타늄 판 위에 제조하여 주사전자현미경을 이용하여 관찰하였다. Figure 1은 전해연마 과정을 통하여 깨끗한 표면의 티타늄 판과 그 표면에 제조된 30, 50, 70 및 100 nm 직경을 가진 티타늄 나노튜브를 보여 주고 있다. 양극산화 전압의 변화에 따른 다양한 직경의 나노튜브가 잘 제조되었음을 알 수 있었다.
2. 다양한 직경의 TiO2 나노튜브에서의 공동배양을 통한 파골세포의 분화 및 생성
공동배양을 통한 TiO2 나노튜브에서의 파골세포의 분화와 생성을 유도하기 위하여 골수세포를 조골세포와 같이 다양한 직경을 가진 TiO2 나노튜브 시편 위에 배양한 후 FDA로 염색하였다. Figure 2A와 B에서 보는 바와 같이 30 nm 직경의 나노튜브 위에서 생성된 파골세포의 수가 전해연마된 티타늄 위에서 보다 2배 정도 많았다. 그러나 나노튜브의 직경이 더 커질수록 생성된 파골세포의 수는 급격하게 줄어들었다. 또한, 전체 TRAP 활성도 30 nm 나노튜브에서 가장 높았고, 직경이 커질수록 현저히 감소되었다(Figure 2C).
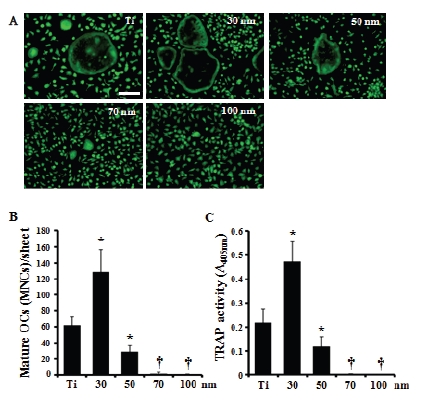
Formation of osteoclast cultured on TiO2 nanotubular structure under coculture system. A-B. Bone marrows were cultured with calvarial osteoblast on the diverse TiO2 nanotubes (30-100 nm) supplemented with L-ascorbic acid (50 μg/ml) and β-glycerophosphate (10 mM). After 9 days, the cells were stained with 2.5% FDA and the cells having diameter lager than 100nm were counted as multinucleated osteoclasts (MNCs). C. Total TRAP activity was measured at an absorbance of 405nm. Data are expressed as the mean±S.D. and are representative of two experiments. Polished Ti sheet was denoted as ‘Ti’. *, p < 0.05 versus Ti, †, p < 0.01 versus Ti. Scale bar = 200nm.
3. 파골세포 전구세포(BMMs) 및 조골세포의 초기 부착능 평가
Figure 3에서 BMMs 및 조골세포의 다양한 직경을 가진 TiO2 나노튜브(30-100 nm)에 대한 부착능을 확인하였다. M-CSF 처리를 통해 골수로부터 유도시킨 BMMs을 48시간 동안 다양한 직경의 나노튜브 위에서 배양해 본 결과 나노튜브 직경과 상관없이 부착된 세포의 수는 유의적 차이가 없었다(Figure 3A, B). 또한 두개골에서 추출한 조골세포의 경우도 72시간 동안 배양하였을 때 BMMs과 마찬가지로 나노튜브 직경과 상관없이 부착된 세포의 수에는 변화가 없었다(Figure 3C, D).
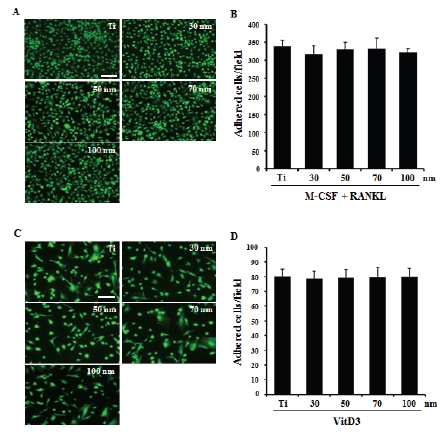
Adhesion of BMMs and calvarial osteoblasts cultured on TiO2 nanotubular structure. A. BMMs were cultured on TiO2 nanotubes (30-100 nm) supplemented with M-CSF for 2 days and were stained with FDA. C. Calvaria osteoblasts were cultured on TiO2 nanotubes (30-100 nm) supplemented with VitD3 (5⨯10-8 M) for 3 days and were stained with FDA. B and D. Adhered cells were counted and expressed as the mean adhered cells±S.D. for one field. Polished Ti sheet was denoted as ‘Ti’. Scale bar = 200 nm.
4. 조골세포의 분화능 평가
조골세포를 다양한 직경의 TiO2 나노튜브에서 9일 동안 분화를 유도하여 조골세포 분화인자(collagen, osteocalcin, osteopontin) 유전자의 발현을 RT-PCR로 측정해 본 결과 Figure 4에서 보는 바와 같이 나노튜브의 직경에 상관없이 모든 유전자의 발현에 차이가 없었다. Osteocalcin (OCN)의 경우 전해연마된 Ti에 비해 나노튜브(30-100 nm)를 가진 TiO2에서 감소되는 경향을 나타내었으나 통계적인 유의차는 측정되지 않았다(p > 0.05).
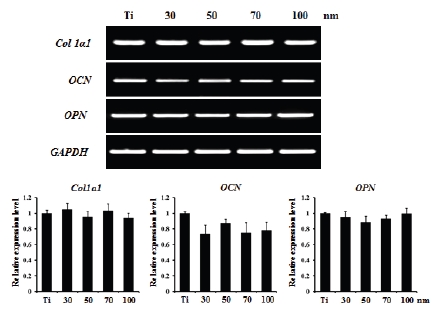
The effect of TiO2 nanotubes on osteoblast differentiation. Calvaria cells were cultured on the diverse TiO2 nanotubes (30-100 nm) supplemented with L-ascorbic acid (50 μg/ml) and β-glycerophosphate (10 mM). After 9 days, the cells were harvested and total RNA was extracted. RT-PCR was performed to detect expression of the indicated genes. Polished Ti sheet was denoted as ‘Ti’.
5. 조골세포에 의한 파골세포 분화유도 인자의 발현 평가
공동배양에서는 조골세포가 분비하는 여러 인자들(M-CSF, RANKL, OPG)이 파골세포의 분화를 조절한다. 따라서 다양한 직경의 TiO2 나노튜브에서의 이러한 파골세포의 분화를 유도하는 인자들의 발현을 확인하였다. 파골세포의 분화와 증식을 조절하는 M-CSF의 경우 TiO2 나노튜브 직경이 커질수록 발현양이 증가되었고, 파골세포 분화에 절대적인 역할을 하는 RANKL의 경우 나노튜브의 직경이 증가될수록 그 발현양은 급격하게 감소되었다(Figure 5A, B). RANKL과 결합할 수 있어 파골세포 분화를 조절하는 decoy receptor로 알려진 OPG의 발현은 나노튜브의 직경이 50 nm까지는 유의적 차이를 보이지 않았지만, 70 nm부터는 그 발현양이 급격하게 증가되었다(Figure 5A, B). 이러한 결과로부터 파골세포 분화와 생성에 영향을 미치는 RANKL/OPG ratio를 비교한 결과, TiO2 나노튜브 직경이 30-50 nm일 때는 전해연마된 티티늄(Ti)와 유의적 차이가 없었지만, 70 nm 나노튜브 직경부터는 그 비율이 급격하게 감소됨을 확인하였다(Figure 5C).
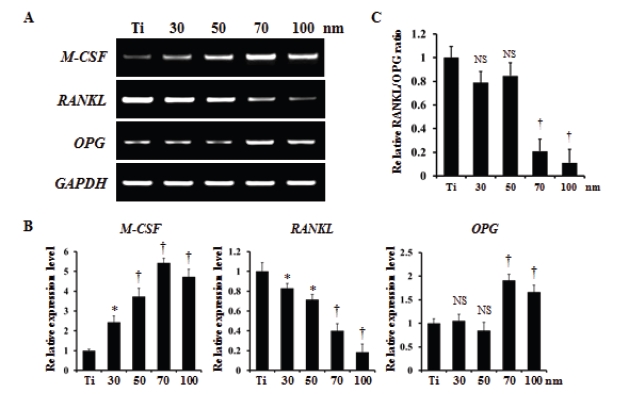
The effect of TiO2 nanotubes on expression of osteoclast differentiation factors. Calvarial cells were cultured on the diverse TiO2 nanotubes (30-100 nm) supplemented with VitD3 (5⨯10-8 M). After 7 days, the cells were harvested and total RNA was extracted. A and B. RT-PCR was performed to detect expression of the indicated genes. C. Relative RANKL/OPG ratio was measured from expression level by RT-PCR. Data are expressed as the mean±S.D. and are representative of two experiments. Polished Ti sheet was denoted as ‘Ti’. *, p < 0.05 versus Ti, †, p < 0.01 versus Ti.
Ⅳ. 고찰
치과 및 정형외과에서는 티타늄(Ti) 또는 티타니아(TiO2) 및 그 합금은 독성이 낮고 내식성이 뛰어나며 기계적 성질이 좋고 생체 적합성이 우수하기 때문에 자주 사용된다. 티타늄은 생불활성(bioinert) 재료이며, 자연산화층이 존재하면 뼈에 결합하는 능력이 증가되고, 전기 화학적 방법을 사용하여 타타늄 표면을 변화시키면 세포와의 상호작용이 향상될 수 있다(Kim and Ramaswamy, 2009; Popat 등, 2007; Bose 등, 2009). 최근, 조골세포(osteoblasts) 및 중간엽줄기세포(MSCs)의 부착, 성장 및 분화에 대한 TiO2 나노튜브의 효과를 평가에 대한 많은 보고가 있다. 여러 연구에서 70-100 nm 직경의 나노튜브에서 배양한 중간엽줄기세포의 골형성 역할을 하는 조골세포로의 분화가 더 증진됨을 보여 주지만(Oh 등, 2009; Wang 등, 2011), 또 다른 연구팀들은 70-100 nm 직경을 가지는 나노튜브가 오히려 세포 사멸을 유도한다고 보고하였다(Park 등, 2007; Von der Mark 등, 2009). 최근, Zhao 등이 세포 배양에서 다른 혈청 농도가 나노튜브의 생물학적 효과 변화를 설명한다고 보고하였지만(Zhao 등, 2012), 아직도 TiO2 나노튜브 직경에 따른 생물학적 효과는 여전히 논쟁거리로 남아 있다. 본 연구에서 다양한 직경(30-100 nm)을 가지는 TiO2 나노튜브 위에 배양한 조골세포 및 파골세포전구세포(BMMs)의 초기 부착능은 나노튜브 직경과 관계없이 동일한 효과를 보였고(Figure 3), 조골세포의 분화에서도 나노튜브 직경에 따른 차이를 확인할 수 없었다(Figure 4). 따라서 나노튜브 직경에 따른 세포의 부착, 증식 및 분화의 효과차이는 여러 가지 실험 조건 등의 차이에 의해 달라질 수 있다고 사료된다.
골유착(osseointegration)의 개념은 임상적 임플란트 분야에서 널리 받아들여지고 있다. 임플란트 표면 형태는 표면에 부착하는 골형성세포(osteoprogenitor cell) 분화와 골기질(bone matrix) 형성을 증진시킴으로서 골유착에 영향을 주는 중요한 요소이다(Dalby et al., 2007). 그러나, 골밀도(bone mass)는 조골세포에 의한 골형성(bone formation)과 파골세포의 골흡수(bone resoption) 활성 사이의 균형에 의해 이루어진다. 파골세포는 인접한 뼈를 재흡수할 수 있을 뿐 아니라 초기 치유(healing) 과정에서 임플란트 표면과 상호 작용할 수 있으며 임플란트의 기능적 수명기간 동안 임플란트 표면에 부착되는 뼈(interfacial bone)의 리모델링에 관여한다(Minkin and Marinho 1999). 또한, 뼈를 흡수하는 파골세포와 뼈 기질을 만드는 조골세포는 생체내 골수(bone marrow)라는 같은 공간에 존재하며 상호작용을 통해 분화와 활성을 조절하고 있을 뿐 아니라 골수 내 다른 세포들과의 상호작용을 통해서 조절을 받고 있다(Walsh 등, 2006). 그러나, 골유착 과정에서 파골세포가 매개하는 골흡수의 중요성은 많이 간과되고 있다.
이전에 본 연구팀은 다양한 직경을 가지는 TiO2 나노튜브 위에 배양한 BMMs을 M-CSF와 RANKL을 처리하여 파골세포로 분화시켰을 때, 나노튜브 직경이 커질수록 파골세포로의 생성이 급격하게 감소함을 보고하였다(Oh and Lee, 2009). 또한, Park 등도 조혈모세포(HSCs)를 M-CSF와 RANKL을 처리하여 파골세포로 분화시킨 연구에서도 동일한 효과를 보고하였다(Park 등, 2009). 그러나 이러한 연구는 파골세포 전구세포 만을 M-CSF와 RANKL 처리하여 파골세포로 분화시킨 실험이다. 따라서 본 연구에서는 생체내 조건과 유사하게 조골세포와 파골세포 전구세포를 다양한 직경을 가지는 TiO2 나노튜브 위에 공동배양하여 나노튜브의 직경이 파골세포 생성에 어떠한 효과를 보이는지 조사하였다. 공동배양에 의한 파골세포 생성은 전해연마된 티타늄보다 30 nm 직경의 TiO2 나노튜브에서 두 배 정도 증가했지만, 50 nm 이상의 직경에서는 급격하게 감소됨을 확인하였다(Figure 2A, B). 전체 TRAP 활성을 측정한 결과에서도 동일한 결과를 보였다(Figure 2C). 따라서 생체내와 유사한 공동배양에서도 TiO2 나노튜브 직경이 증가할수록 파골세포의 분화 및 생성이 억제됨을 알 수 있다.
파골세포 형성(osteoclatogenesis)은 분화하는 조골세포에서 분비하는 RANKL 및 OPG에 의해 직접적으로 영향을 받는다. 임플란트 표면 형태는 조골세포가 생성하는 RANKL와 OPG 같은 파골세포생성과 관계된 많은 골관련(bone-related) 단백질 발현에 영향을 미친다(Nagasawa 등, 2015). 따라서, 본 연구팀은 공동배양을 통한 파골세포 생성에서 TiO2 나노튜브 직경에 따른 억제 효과의 기전에 대해 조사하였다. 다양한 직경의 TiO2 나노튜브 위에 배양한 조골세포가 분비하는 파골세포 분화 관련 단백질 발현양상을 평가하였다. Figure 5에서 보는 바와 같이 M-CSF와 OPG의 발현은 나노튜브의 사이즈가 커질수록 증가하였고, 특히 70 nm일 때 최대치를 보였고, RANKL의 발현은 나노튜브의 사이즈가 커질수록 감소하며 100 nm일 때 최저치를 보인다는 것을 확인하였다. 또한, 조골세포에 의한 파골세포 생성에 중요한 인자인 RANKL/OPG ratio가 나노튜브 직경이 70-100 nm일 때 가장 감소함을 알 수 있었다. 이상의 결과를 종합해 볼 때 골수세포와 조골세포의 공동배양 시 TiO2 나노튜브 직경이 커질수록 파골세포의 생성이 감소하는 이유는 세포들의 부착능 및 조골세포의 분화능의 차이가 아니라 RANKL 및 OPG를 분비하여 파골세포 분화를 이끄는 조골세포 기능의 감소 때문이라고 유추할 수 있다. 따라서, TiO2 나노튜브 직경이 70-100 nm일 때 임플란트 주변의 골유착에 있어서 파골세포에 의한 골흡수 문제를 최소화할 수 있을 것이라고 판단된다. 이러한 결과는 70-100 nm 직경의 나노튜브에서 중간엽줄기세포의 조골세포로의 분화가 더 증진됨을 보여 준 이전의 연구(Oh et al., 2009; Wang et al., 2011)와 더불어 70-100nm 직경의 TiO2 나노튜브의 임상적 적용 가능성을 제시한다.
Ⅴ. 결론
본 연구에서는 전해연마된 티타늄 위에 제조된 30-100 nm 직경의 TiO2 나노튜브에 골수세포와 조골세포를 공동배양 하였을 때, 다양한 직경을 가진 나노튜브의 파골세포 분화와 생성에 미치는 효과와 그 기전을 평가하여 다음과 같은 결과를 얻었다.
1. 골수세포와 조골세포를 공동배양하였을 때 파골세포의 생성은 나노튜브의 직경이 30 nm 일 때 가장 증가되었고, 그 이상의 직경을 가진 나노튜브에서는 급격하게 감소되었다.
2. 파골세포 전구세포 및 조골세포의 초기 부착능을 측정한 결과 나노튜브 직경에 상관없이 각 세포의 부착능에는 유의적 차이를 보이지 않았다(p > 0.05).
3. 조골세포의 분화 인자들(Collagen type1 alpha 1 (Col1α1), osteocalcin (OCN), osteopontin (OPN))의 발현을 측정한 결과 나노튜브의 직경에 상관없이 측정한 모든 인자들의 발현은 유의적 차이를 보이지 않았다(p > 0.05).
4. 조골세포가 분비하는 파골세포 분화유도 인자(M-CSF, OPG, RANKL)의 발현을 확인한 결과 M-CSF와 OPG는 나노튜브의 직경이 커질수록 그 발현양이 증가되는 경향을 보였고, 직경이 7 0nm일 때 가장 발현양이 증가됨을 보였다. 그러나 RANKL의 경우 나노튜브의 직경이 커질수록 그 발현양이 현저히 감소됨을 보였다. 또한 RANKL/OPG ratio는 나노튜브 직경이 70 nm일 때부터 급격히 감소되었다.
5. 이상의 연구의 결과로 나노튜브의 직경이 70-100 nm 일 때 조골세포의 부착능과 분화에는 영향을 주지 않고 파골세포의 생성을 가장 잘 억제할 수 있다고 판단된다. 따라서 임상적 적용에 있어서 티타늄 임플란트의 표면에 70-100 nm 직경의 나노튜브를 제작하는 것이 임플란트의 효과를 극대화시킬 수 있을 것이라 사료된다.
Acknowledgments
이 논문은 2016년도 원광대학교 교내연구비 지원에 의한 논문임.
These authors contributed equally to this work.
References
- Albrektsson, T, Wennerberg, A, (2005), The impact of oral implants—past and future, 1966–042, 042. J Canadian Dental Association, 71, p327-327.
-
Bertoncini, P, Le Chevalier, S, Lavenus, S, Layrolle, P, Louarn, G, (2012), Early adhesion of human mesenchymal stem cells on TiO2 surfaces studied by single-cell force spectroscopy measurements, J. Mol. Recognit, 25, p262-269.
[https://doi.org/10.1002/jmr.2193]
-
Brammer, KS, Oh, S, Cobb, CJ, Bjursten, LM, Heyde, HVD, Jin, S, (2009), Improved bone-forming functionality on diameter controlled TiO2 nanotube surface, Acta Biomaterialia, 5, p3215-3223.
[https://doi.org/10.1016/j.actbio.2009.05.008]
-
Brammer, KS, Oh, S, Gallagher, JO, Jin, S, (2008), Enhanced cellular mobility guided by TiO2 nanotube surfaces, Nano Letters, 8, p786-793.
[https://doi.org/10.1021/nl072572o]
-
Bose, S, Roy, M, Das, K, Bandyopadhyay, A, (2009), Surface modification of titanium for load-bearing applications, J Mater Sci Mater Med, 20, pS19-S24.
[https://doi.org/10.1007/s10856-008-3418-1]
-
Dalby, MJ, Gadegaard, N, Tare, N, Andar, A, Riehle, MO, Herzyk, P, Wilkinson, CDW, Oreffo, ROC, (2007), The control of human mesenchymal cell differentiation using nanoscale symmetry and disorder, Nat Mater, 6, p997-1003.
[https://doi.org/10.1038/nmat2013]
-
Hofbauer, LC, Dunstan, CR, Spelsberg, TC, Riggs, BL, Khosla, S, (1998), Osteoprotegerin production by human osteoblast lineage cells is stimulated by vitamin D, bone morphogenetic protein-2, and cytokines, Biochem Biophys Res Commun, 250, p776-781.
[https://doi.org/10.1006/bbrc.1998.9394]
-
Hofbauer, LC, Lacey, DL, Dunstan, CR, Spelsberg, TC, Riggs, BL, Khosla, S, (1999), Interleukin-1beta and tumor necrosis factor-alpha, but not interleukin-6, stimulate osteoprotegerin ligand gene expression in human osteoblastic cells, Bone, 25, p255-259.
[https://doi.org/10.1016/S8756-3282(99)00162-3]
-
Huang, JC, Sakata, T, Pfleger, LL, Bencsik, M, Halloran, BP, Bikle, DD, Nissenson, RA, (2004), PTH differentially regulates expression of RANKL and OPG, J Bone Miner Res, 19, p235-244.
[https://doi.org/10.1359/JBMR.0301226]
-
Kim, JH, Kim, N, (2014), Regulation of NFATc1 in osteoclast differentiation, J Bone Metab, 21, p233-241.
[https://doi.org/10.11005/jbm.2014.21.4.233]
-
Kim, KH, Ramaswamy, N, (2009), Electrochemical surface modification of titanium in dentistry, Dent Mater J, 28, p20-36.
[https://doi.org/10.4012/dmj.28.20]
-
Le Gu´ehennec, L, Soueidan, A, Layrolle, P, Amouriq, Y, (2007), Surface treatments of titanium dental implants for rapid osseointegration, Dental Materials, 23, p844-854.
[https://doi.org/10.1016/j.dental.2006.06.025]
- Linder, L, Carlsson, A, Marsal, L, Bjursten, LM, Branmartk, PI, (1988), Clinical aspects of osseointegration in joint replacement, J Bone Joint Surg, 70-B, p550-555.
-
Minkin, C, Marinho, VC, (1999), Role of the osteoclast at the bone-implant interface, Adv Dent Res, 13, p49-56.
[https://doi.org/10.1177/08959374990130011401]
-
Nagasawa, M, Cooper, LF, Ogino, Y, Mendonca, D, Liang, R, Yang, S, Mendonca, G, Uoshima, K, (2015), Topography Influences Adherent Cell Regulation of Osteoclastogenesis, J. Dent. Res, 95, p319-326.
[https://doi.org/10.1177/0022034515616760]
-
Oh, S, Brammer, KS, Li, YSJ, Teng, D, Engler, AJ, Chien, S, Jin, S, (2009), Stem cell fate dictated solely by altered nanotube dimension, Proc Natl Acad Sci U.S.A., 106, p2130-2135.
[https://doi.org/10.1073/pnas.0813200106]
-
Oh, S, Daraio, C, Chen, LH, Pisanic, TR, Finones, RR, Jin, S, (2006), Significantly accelerated osteoblast cell growth on aligned TiO2 nanotubes, J Biomed Mater Res A, 78, p97-103.
[https://doi.org/10.1002/jbm.a.30722]
- Oh, S, Lee, SH, (2009), Ability of Osteoclasts Adhesion and Differentiation on Various Pore Scale of TiO2 Nanotube Surface, Kor J Dent Mater, 36, p315-320.
-
Park, J, Bauer, S, Schlegel, KA, Neukam, FW, Von Mark, KD, Schmuki, P, (2009), TiO2 nanotube surfaces: 15nm—an optimal length scale of surface topography for cell adhesion and differentiation, Small, 5, p666-671.
[https://doi.org/10.1002/smll.200801476]
-
Park, J, Bauer, S, von der Mark, K, Schmuki, P, (2007), Nanosize and vitality: TiO2 nanotube diameter directs cell fate, Nano Letters, 7, p1686-1691.
[https://doi.org/10.1021/nl070678d]
-
Popat, KC, Leoni, L, Grimes, CA, Desai, TA, (2007), Influence of engineered titania nanotubular surfaces on bone cells, Biomaterials, 28, p3188-3197.
[https://doi.org/10.1016/j.biomaterials.2007.03.020]
-
Satomi, K, Alagawa, Y, Nikai, H, Tsuru, H, (1988), Bone-implant interface structures after nontapping and tapping insertion of screw-type titanium alloy endosseous implants, J Prosthet Dent, 59, p339-342.
[https://doi.org/10.1016/0022-3913(88)90187-4]
-
Takayanagi, H, (2007), Osteoimmunology: shared mechanisms and crosstalk between the immune and bone systems, Nat Rev Immunol, 7, p292-304.
[https://doi.org/10.1038/nri2062]
-
Von der Mark, K, Bauer, S, Park, J, Schmuki, P, (2009), Another look at Stem cell fate dictated solely by altered nanotube dimension, Proc Natl Acad Sci U.S.A., 106, pE60.
[https://doi.org/10.1073/pnas.0903663106]
- Walsh, MC, Choi, Y, (2014), Biology of the RANKLRANK-OPG System in Immunity, Bone, and Beyond, Front Immunol, 5-511, p1-11.
-
Walsh, MC, Kim, N, Kadono, Y, Rho, J, Lee, SY, Lorenzo, J, Choi, Y, (2006), Osteoimmunology: interplay between the immune system and bone metabolism, Annu Rev Immunol, 24, p33-63.
[https://doi.org/10.1146/annurev.immunol.24.021605.090646]
-
Wang, N, Li, H, Lü, W, Li, J, Wang, J, Zhang, Z, Liu, Y, (2011), Effects on TiO2 nanotubes with different diameters on gene expression and osseointegration of implants in minipigs, Biomaterials, 32, p6900-6911.
[https://doi.org/10.1016/j.biomaterials.2011.06.023]
-
Zhao, L, Liu, L, Wu, Z, Zhang, Y, Chu, PK, (2012), Effects on micropitted/nanotubular titania topographies on bone mesenchymal stem cell osteogenic differentiation, Biomaterials, 32, p2629-2641.
[https://doi.org/10.1016/j.biomaterials.2011.12.024]