
Evaluation of corrosion resistance of stainless steel dental hand instruments using salt spray testing
Abstract
With advancements in the materials and design of sinus membrane elevators, sinus lift surgery has become a commonly performed procedure even in general outpatient clinics. Sinus membrane elevators currently available on the markets are made of 304 and 420 stainless steel (SS), which exhibit high corrosion resistance due to their austenitic or martensitic structures. For these instruments to be commercially viable, they must not show any visible signs of corrosion after 100 autoclave cycles. However, from a manufacturer’s perspective, this verification method is time–consuming and resource–intensive. The salt spray test is an industry method used to accelerate corrosion in materials and assess their corrosion resistance. In this study, we fabricated standard specimens of 304 and 420 SS and demonstrated that the level of corrosion equivalent to 100 autoclave cycles was observed after 60 min of salt spray testing. Based on these findings, we collected 15 types of sinus membrane elevators available on the market and subjected them to salt spray testing. As a result, 14 out of 15 instruments passed the 60 min salt spray test. These results suggest the possibility of replacing 100 autoclave cycles with 60 min of salt spray testing.
초록
상악동막 거상기의 소재 및 디자인 발전으로 상악동막 거상술은 일반 외래에서도 흔하게 시행되는 수술이 되었다. 시중에 판매되는 상악동 막 거상기는 304 그리고 420 스테인리스강으로 구성되어 있으며, 이 재료들은 오스테나이트 또는 마르텐사이트 구조를 가져 높은 부식저항성을 보인다. 이 기구들이 시판되려면 100회 오토클레이브를 반복해도 육안으로 관찰했을 때 부식을 보여선 안된다. 하지만 이러한 검증법은 제조사 입장에서 많은 시간과 재원이 필요한 까다로운 방법이다. 염수분무실험은 재료의 부식을 가속화 시키는 방법으로 산업계에서 부식저항성을 측정하는데 사용된다. 본 연구에서는 304 그리고 420 스테인리스강 기준시편을 제작해 100회 오토클레이브에 해당하는 부식 정도가 60분 염수 분무 실험에서 나타났음을 밝혔다. 이를 토대로 시장에서 유통되고 있는 15종의 상악동 막 거상기를 수집하여 염수분무 실험을 진행한 결과 14종의 기구가 60분 염수분무 실험을 통과하였다. 이로서 오토클레이브 100회 반복을 염수분무 60분으로 대체할 수 있는 가능성을 제시했다.
Keywords:
Stainless steel, Sinus membrane elevator, Autoclave, Salt spray test, Corrosion resistance키워드:
상악동막 거상기, 오토클레이브, 염수분무실험, 부식저항성Introduction
Sinus lift surgery is a procedure designed to increase maxillary sinus bone volume by elevating the Schneiderian membrane and inserting a bone graft (1). This membrane elevation is facilitated using a sinus membrane elevator (2). According to ISO 19490, a sinus membrane elevator is a dental instrument employed during implant placement to elevate the sinus floor, thereby increasing vertical bone thickness (3). The elevator typically consists of three primary components: the handle, shank, and working end (4). ISO 21850–1 specifies that sinus membrane elevators should be manufactured using 420 stainless steel (SS) (5), a high–carbon martensitic steel containing at least 12% chromium (6). It is well–established that a minimum of 11% chromium is necessary to achieve corrosion resistance through the formation of a protective oxide layer. However, prior research has demonstrated that martensitic SS, such as 420 SS, is prone to localized corrosion in chloride–rich environments, particularly marine conditions (7). In addition to 420 SS, 304 SS is often used in the fabrication of the handle portion of sinus membrane elevators. 304 SS, the most widely utilized austenitic SS, contains 18–20% chromium and 8–10.5% nickel (6). Despite its widespread use, 304 SS is also susceptible to pitting and crevice corrosion in warm chloride environments and can experience stress corrosion cracking at temperature above 60 ℃ (8).
ISO 4865–1 mandates that non–hinged instruments, including sinus membrane elevators, must withstand 100 autoclave cycles without visible corrosion when inspected visually (9). Furthermore, these instruments are required to maintain their microhardness throughout the repeated sterilization processes. As a critical invasive instrument among dental hand instruments, the sinus membrane elevator plays a significant role in patient safety. Corrosion of this instrument poses a potential risk to patients. Therefore, the ISO guidelines stipulate stringent evaluation protocols to ensure corrosion resistance and prevent adverse incidents.
From a manufacturing perspective, adhering to this protocol can be challenging, as each instrument must be inspected for corrosion or surface defects and undergo microhardness testing after every cycle during the 100 cycles evaluation. Additionally, the American Dental Association recommends that, following sinus lift surgery, used sinus membrane elevators should be cleaned with a disinfecting detergent and scrubbed. This disinfecting detergent often contains sodium hypochlorite (NaOCl) to remove absorbed proteins from the instrument’s surface (10), exposing the elevator to chlorides. In contrast, the autoclave sterilization process involves only distilled water, leading to a significant discrepancy between clinical practice and ISO standards.
ISO 9227 outlines guidelines for the salt spray test, a method designed to compare the corrosion resistance of metallic materials (11). The salt spray test creates an accelerated corrosion environment on metal surface and has been widely used in prior studies to assess the corrosion resistance of stainless steel (12–14). However, no research has attempted to correlate the salt spray test with repetitive autoclave cycles to identify a similar magnitude of corrosion. This study aims to determine the optimal salt spray test duration that could serve as an alternative to 100 autoclave cycles for evaluating the sinus membrane elevator.
Materials and Methods
1. Preparation of standard 304 and 420 stainless steel specimen and 15 commercial sinus membrane elevators
Cylinder raw materials (5 mm in diameter) of 304 and 420 SS were obtained from the manufacturer (OSUNG MND, Gimpo, Gyeonggi–do, Korea). These materials were divided into two groups: one group was used directly in the experiments, while the other underwent heat treatment. The heat treatment conditions were as follows: For 304 SS, specimens were heated to 1050 ℃, held for 2 h, and then quenched. For 420 SS, specimens were heated to 1030 ℃, held for 2 h, and quenched, and then tempered at 260 ℃ for 4 h.
Additionally, 15 different commercial sinus membrane elevators were prepared for analysis. The working ends and handles of each sinus membrane elevator were sectioned and embedded in epoxy resin. The specimens were sequentially ground suing SiC papers (#240, 320, 400, and 600) and polished using a 6 µm diamond paste followed by a 0.05 µm alumina (Al2O3) suspension. After each grinding step, the specimens were ultrasonically cleaned for 3 min to remove debris. Specimens were etched for 10 s in 2% nital, rinsed with distilled water and ethanol, and air–dried at room temperature. The etched surface were examined three times using: a metallographic microscope (MM–11; Nikon, Minato City, Tokyo, Japan), a scanning electron microscope (SEM; IT–500HR, JEOL, Akishima, Tokyo, Japan), and energy dispersive X–ray spectroscopy (EDS). Table 1 summarizes the company names, countries of origin, and materials used for the working ends and handles of the elevators.
2. Microhardness and microstructure analysis of standard specimens after repeated autoclave cycles
Four groups of specimens were prepared: 304 SS_heat treatment and 304 SS_non–heat treatment, 420 SS_heat–treatment and 420 SS_non–heat treatment. Vickers hardness testing was conducted (MMT–X; Matsuzawa, Akita, Japan), and microstructural analysis was performed at 500× magnification after 0, 20, 40, 60, 80, and 100 autoclave cycles.
3. Evaluation of corrosion levels of standard specimen in relation to the autoclave cycles and salt spray exposure
For corrosion analysis through visual inspection, two experts participated. Corrosion was confirmed on a specimen when both experts agreed on its occurrence.
Each specimen was individually sealed in an autoclave pouch and sterilized in an autoclave set to 121 ℃ for 15 min using distilled water. Repeated autoclave cycles were conducted for 20, 40, 60, 80, and 100 cycles. For the salt spray test, NaCl was dissolved in distilled water to achieve a solution density of 1.023–1.036 g/cm3 with a pH of 6.5–7.2. The chamber air pressure was maintained at 0.07–0.17 MPa, and the temperature was set at 35 ℃. Salt spray exposure times were 30, 60, 90, and 120 min. After testing, specimens were cleaned with distilled water, dried using N2 gas, and examined using SEM to evaluate corrosion levels.
4. Evaluation of microhardness of 15 commercial sinus membrane elevators following salt spray testing
The microhardness of the 15 different sinus membrane elevators was evaluated before and after salt spray testing. Measurements were taken after 30, 60, 90, and 120 min of exposure.
5. Evaluation of corrosion in relation to salt spray exposure duration
15 different sinus membrane elevators were subjected to salt spray testing for 30, 60, 90, or 120 min. After each time point, the samples were removed, washed, dried, and inspected for corrosion using visual examination and SEM.
Results
1. Analysis of materials for the working end and handle of sinus membrane elevators
Analysis of 15 different sinus membrane elevators revealed that the working ends of 10 out of the 15 instruments were composed of 420 SS, while the handles of 11 instruments were made from 304 SS.
2. Mechanical characteristics of 304 and 420 SS standard specimens following repetitive autoclave treatment cycles
The microhardness results are presented in Figure 1. Heat treatment led to a decrease in the microhardness of 304 SS, while the microhardness of 420 SS increased post–treatment. Microstructural analysis, shown in Figure 2, indicated no significant changes with respect to the number of autoclave cycles. Before heat treatment, the grains of 304 SS exhibited slip bands, indicative of plastic deformation. After heat treatment, no slip bands were observed, and the grains displayed an austenitic structure. In 420 SS, numerous unutilized carbides were present, leading to lower microhardness; however, heat treatment transformed the microstructure into a martensitic structure.
3. Corrosion levels of standard specimens in relation to autoclave cycles and salt spray duration
SEM based corrosion analysis revealed differences in corrosion resistance among the specimens, as shown in Figure 3. The 304 SS_Heat treatment group exhibited no signs of corrosion after 100 autoclave cycles and 120 min of salt spray exposure, demonstrating high corrosion resistance. In contrast, the 304 SS_Non–heat treatment group showed corrosion after 100 autoclave cycles and 60 min of salt spray exposure, indicating lower resistance. The 420 SS specimens demonstrated overall lower corrosion resistance compared to 304 SS. Corrosion was observed in the 420 SS_Heat treatment group after 100 autoclave cycles and 60 min of salt spray exposure. Furthermore, the 420 SS_Non–heat treatment group exhibited pitting corrosion after 100 autoclave cycles and 90 min of salt spray exposure.
In summary, the corrosion magnitude observed after100 autoclave cycles was equivalent to 60–90 min of salt spray exposure, as evidenced by the data in Figure 4 and 5. The 420 SS heat–treatment group showed corrosion after 100 autoclave cycles, and during the salt spray test, corrosion was detected 60 min via SEM analysis. Visual inspection results (Figure 5) revealed a similar trend to the SEM results shown in Figure 4.
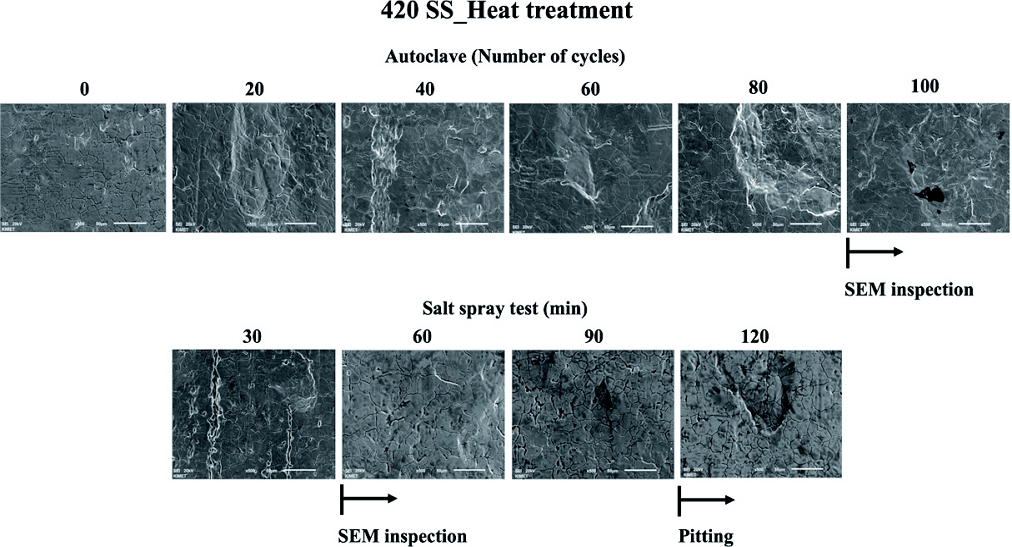
SEM images of 420 SS_Heat treatment, showing the effects of the number of autoclave cycles and the duration of salt spray exposure.
4. Evaluation of microhardness of 15 different type of sinus membrane elevator
The microhardness of the sinus membrane elevators remained unchanged after autoclave and salt spray testing, as shown in Figure 5. This indicates that the initial microhardness of the instruments was preserved. The minimum acceptable microhardness for sinus membrane elevators, as indicated by red dotted line in Figure 5, is 480 HV. However, three instruments – Hu–Firedy (440A SS), Dental Studio (Ni–Ti), MCT Bio (304 SS) did not meet this requirement.
5. Corrosion detection based on salt spray duration
In Figure 7, visual inspection revealed no corrosion in any instruments after 30 min of salt spray testing. Since all instruments met the ISO 19490 corrosion resistance standard, a 30 min salt spray test is considered equivalent to under 100 autoclave cycles. At 60 min, corrosion was visually observed in one instrument (I–dent). The I–dent elevator, primarily composed of 420 SS, contained inclusions of aluminum and calcium, which likely contributed to its corrosion. This anomaly was excluded when determining the optimal salt spray test duration. SEM analysis revealed that only three instruments–Dental Studio, BERA Dental, and OSUNG–showed no signs of corrosion even after extended testing. Dental Studio's working end is composed of Ni–Ti with an electrolytic polished surface, OSUNG has a TiN coating, and BERA Denal has a Ti–Cr coating. These surface treatments were effective in preventing corrosion. Conversely, 5 instruments – Dental USA, Young Dent, Surgident, I–Dent, and Atira – exhibited corrosion after the 30 min salt spray test. A common characteristic among these instruments was high surface roughness, which was associated with increased susceptibility to corrosion.
Discussion
This research represents the first attempt to analyze the relationship between salt spray test duration and the number of autoclave cycles. While the salt spray test is already established for evaluating the corrosion resistance of metallic materials according to ISO 10271, the evaluation of dental instruments typically relies on 100 autoclave cycles to assess their corrosion resistance.
The microhardness and microstructure of 304 and 420 SS standard specimens remained consistent across varying numbers of autoclave cycles (Figure 1 and 2). Although corrosion was observed in non–heat treatment groups, their microhardness values were unaffedcted. These findings suggest that 100 repetitive autoclave cycles do not alter the microstructure or microhardness of 304 and 420 SS.
Heat treatment significantly impacts corrosion resistance. 304 SS_ and 420 SS_ Heat treatment groups exhibited greater corrosion resistance compared to non–heat treatment specimens due to microstructural change introduced during heat treatment. Despite this improvement, the 420 SS_heat–treatment group still displayed corrosion after 100 autoclave cycles (Figure 3), likely attributable to the high surface roughness of the specimens. Surface roughness is a critical determinant of corrosion resistance, with higher roughness levels increasing susceptibility to corrosion (15). In contrast, commercial sinus membrane elevators, typically composed of 304 and 420 SS, are manufactured with smoother surfaces, which enhances their resistance to corrosion. Consequently, these instruments maintained both their microhardness and microstructure after 100 repetitive autoclave cycles.
In the pilot study, a 120 min salt spray test induced corrosion in most dental instruments, such as explorers. To identify the initial corrosion point, we selected 30, 60, 90, and 120 min as the duration for the salt spray test. The 304 and 420 SS standard specimens serve as suitable models for sinus membrane elevators and provide a foundation for correlating autoclave cycles with salt spray test duration (Figure 3). Austenitic steel, such as 304 SS_heat–treatment, is known for their superior corrosion resistance compared to martensitic steels (16). In this study, the 304 SS_heat–treatment group exhibited no corrosion under either autoclave or salt spray conditions. Conversely, non–heat treated 304 SS and 420 SS specimens demonstrated surface corrosion in both tests. SEM analysis revealed comparable corrosion levels between 100 autoclave cycles and 60 min of salt spray exposure. This finding suggests that the salt spray test can replicate the effects of repeated autoclave cycles. ISO 19490 specifies that no corrosion should be visually detectable after 100 autoclave cycles. However, ISO 10271 extends the evaluation criteria by incorporating SEM analysis, which can detect early–stage corrosion not visible to the naked eye (17). SEM analysis also provide detailed insights into corrosion types, such as pitting, and reveals microstructural changes in specimens. The combination of the salt spray test with SEM inspection addresses the limitation of ISO 19490, particularly regarding the reliance on visual inspection and its time–intensive nature.
The relationship between corrosion resistance and microhardness in martensitic materials has been debated. While some studies suggest a correlation (18–20), others present counterexamples (21). Our findings align with the latter perspective, indicating that microhardness alone does not predict corrosion resistance. For instance, the highest microhardness values were observed in the Surgident and I–dent sinus membrane elevators, yet these instruments exhibited poor corrosion resistance (Figure 7). Notably, the I–dent elevator showed visible corrosion after just 30 min of salt spray exposure. Thus, initial microhardness cannot be considered a reliable predictor of corrosion resistance.
Despite its advantages, the salt spry test has limitations when evaluating the corrosion resistance of sinus membrane elevators. First, the position and orientation of the instruments in the salt spray chamber can affect results, as the distribution of the spray may not be uniform. Future studies should address this limitation by ensuring homogeneous salt spray distribution. Second, the unpredictable nature of corrosion introduces variability, with factors such as surface defects potentially leading to inconsistent results. Secondly, corrosion is difficult to predict, and numerous factors can influence the outcome of corrosion tests. For example, surface defects on the instruments can lead to unexpected corrosion. Despite these limitations, the salt spray test offers a significantly shorter testing time compared to autoclave cycles, potentially reducing the instruments’ exposure to harmful factors. Therefore, 100 autoclave cycles could be replaced by 60 min of salt spray testing.
Conclusion
The traditional method of assessing corrosion resistance in sinus membrane elevator through repetitive autoclave cycles can be replaced with the more time and energy efficient salt spray test. This study demonstrated that 100 autoclave cycles and a 60 min salt spray test produce comparable levels of corrosion on standard specimens, such as 304 and 420 SS. Therefore, a sinus membrane elevator that successfully withstands a 60 min salt spray test without showing corrosion is expected to meet the visual inspection criteria for corrosion resistance.
Acknowledgments
This work was supported by the Korea Medical Device Development Fund grant funded by the Korea government (the Ministry of Science and ICT, the Ministry of Trade Industry and Energy the Ministry of Health & Welfare the Ministry of Food and Drug Safety) ((Project Number: RS-2023-00210701)
References
-
Boyne PJ, Lilly LC, Marx RE, Moy PK, Nevins M, Spagnoli DB, et al. De novo bone induction by recombinant human bone morphogenetic protein-2 (rhBMP-2) in maxillary sinus floor augmentation. J Oral Maxillofac Surg. 2005;63(12):1693-707.
[https://doi.org/10.1016/j.joms.2005.08.018]
-
Stacchi C, Lombardi T, Ottonelli R, Berton F, Perinetti G, Traini T. New bone formation after transcrestal sinus floor elevation was influenced by sinus cavity dimensions: A prospective histologic and histomorphometric study. Clin Oral implants Res. 2018;29(5):465-79.
[https://doi.org/10.1111/clr.13144]
- International Organization for Standardization. ISO 19490:2017. Dentistry – Sinus membrane elevator. Geneva: ISO;2017
- Jensen OT, Shulman LB, Block MS, Iacono V. Report of the Sinus Consensus Conference of 1996. Int J Oral Maxillofac implants. 1998;13:11-45.
- International Organization for Standardization. ISO 21850-1:2020. Dentistry – Materials for dental instruments. Geneva: ISO;2020
- Candelaria A, Pinedo C. Influence of the heat treatment on the corrosion resistance of the martensitic stainless steel type AISI 420. J Mater Sci. 2003;22(16):1151-3.
-
Donahue JR, Burns JT. Effect of chloride concentration on the corrosion–fatigue crack behavior of an age-hardenable martensitic stainless steel. Int J Fatigue. 2016;91:79-99.
[https://doi.org/10.1016/j.ijfatigue.2016.05.022]
-
Chou W-J, Yu G-P, Huang J-H. Corrosion behavior of TiN-coated 304 stainless steel. Corros Sci. 2001;43(11):2023-35.
[https://doi.org/10.1016/S0010-938X(01)00010-5]
- International Organization for Standardization. ISO 4865-1:2023. Dentistry – General requirements of hand instruments. Geneva: ISO;2023
-
Ziemniak S, Hanson M. Corrosion behavior of 304 stainless steel in high temperature, hydrogenated water. Corros Sci. 2002;44(10):2209-30.
[https://doi.org/10.1016/S0010-938X(02)00004-5]
-
Alleng G. Enzymatic detergent use for gastroscope cleaning, surgical hand disinfectants, instument decontamination, residual protein levels. AORN J. 2006;84(5):863-9.
[https://doi.org/10.1016/S0001-2092(06)63973-2]
-
Dobrzański LA, Brytan Z, Grande MA, Rosso M. Corrosion resistance of sintered duplex stainless steels in the salt fog spray test. J Mater Process Technol. 2007;192:443-8.
[https://doi.org/10.1016/j.jmatprotec.2007.04.077]
-
Olugbade TO, Omiyale BO. Corrosion resistance of surface-conditioned 301 and 304 stainless steels by salt spray test. Anal Tech Szeged. 2021;15(2):9-19.
[https://doi.org/10.14232/analecta.2021.2.9-19]
-
Chou WJ, Yu GP, Huang JH. Corrosion behavior of TiN-coated 304 stainless steel. Corros Sci. 2001;43(11):2023-35.
[https://doi.org/10.1016/S0010-938X(01)00010-5]
-
Hilbert LR, Bagge-Ravn D, Kold J, Gram L. Influence of surface roughness of stainless steel on microbial adhesion and corrosion resistance. Int Biodeter Biodegr. 2003;52(3):175-85.
[https://doi.org/10.1016/S0964-8305(03)00104-5]
-
Abreu HF, Carvalho SS, Lima Neto PD, Santos RP, Freire VN, Silva PM, Tavares SS. Deformation induced martensite in an AISI 301LN stainless steel: characterization and influence on pitting corrosion resistance. Mater Res. 2007;10:359-66.
[https://doi.org/10.1590/S1516-14392007000400007]
-
Pantelis DI, Bouyiouri E, Kouloumbi N, Vassiliou P, Koutsomichalis A. Wear and corrosion resistance of laser surface hardened structural steel. Surf Coat Technol. 2002;161(2-3):125-34.
[https://doi.org/10.1016/S0257-8972(02)00495-4]
-
Bordbar S, Alizadeh M, Hashemi SH. Effects of microstructure alteration on corrosion behavior of welded joint in API X70 pipeline steel. Mater Des. 2013;45:597-604.
[https://doi.org/10.1016/j.matdes.2012.09.051]
- International Organization for Standardization. ISO 10271:2020. Dentistry – Corrosion test methods for metallic materials. Geneva: ISO;2020
-
Alizadeh M, Bordbar S. The influence of microstructure on the protective properties of the corrosion product layer generated on the welded API X70 steel in chloride solution. Corros Sci. 2013;70:170-9.
[https://doi.org/10.1016/j.corsci.2013.01.026]
-
Moura VS, Lima LD, Pardal JM, Kina AY, Corte RR, Tavares SS. Influence of microstructure on the corrosion resistance of the duplex stainless steel UNS S31803. Mater Charact. 2008;59(8):1127-32.
[https://doi.org/10.1016/j.matchar.2007.09.002]