
Influence of storage media on degradation of dental composite resins
초록
이 연구에서는 세 가지 다른 보관 용액이 두 복합 레진의 화학적 열화에 미치는 영향을 Knoop 경도, 표면 조도, 레진 재료의 무게 변화로 평가하였다. Microfilled type의 복합 레진인 Esthet·X와 Clearfil AP-X를 본 실험에 사용하였다. 위 아래 면을 각각 40초 광조사한 레진 시편을 37℃ 증류수에서 24시간 보관한 후 Knoop 경도, 표면 조도, 무게의 초기 값을 측정하였다. 그 후 시편을 pH-cycling, HCl(pH 4.0±0.1), 증류수 중 한 용액(37℃)에 보관하였다(n=5). 3일, 1주, 2주 시편을 보관 후, 위의 값을 측정하여 초기 값과 비교하였고, 2주 보관 후의 시편의 표면 상태를 주사전자현미경으로 관찰하였다. 보관용액에 따른 측정 값의 유의한 차이는 발견되지 않았지만, 산 용액은 pH-cycling과 증류수에 비해 더 많은 열화를 보였다
Keywords:
Degradation, pH-cycling, Knoop hardness, Surface roughnessⅠ. INTRODUCTION
Composite resins are currently one of the most widely used in restorative dentistry and the satisfactory clinical performance is largely determined by its resistance to degradation in the oral environment. Many polymer networks are considered to be largely insoluble structures with relatively high chemical and thermal stability (Ferracane 2006). However, the networks may absorb water and chemicals from any environment and can cause degradation of the polymers (Ferracane 2006).
Corrosive wear of composites results from the joint action of chemical and mechanical forces, and is associated with the mechanical removal of corroded layers that form on the surface of a material by reaction with its environment (Sarkar 2000). However, Roulet & Walti (1984) and van Groeningen et al. (1986) showed that the degradation of composites in the oral environment may occur even in the absence of loading and abrasive forces. Mechanical testing performed in slightly lower pH environment can simulate clinical situations better, compared to a condition in water (Prakki et al. 2005).
One of the important physical properties of restorative materials is surface hardness or roughness. Chemical environment may play an important role in influencing hardness, surface roughness or weight of composites. Measuring hardness of composites is an indirect method for evaluating the mechanical strength and resistance to intraoral softening of the materials (Watts et al. 1987). Even short duration of immersion will influence mechanical wear. The resistance to initial softening will improve the abrasion resistance of dental composite restorations (Wu & McKinney 1982). And surface roughness is important in applications involving friction, lubrication, and wear (Thomas 1998). The surface roughness of composites may be significantly affected by both water absorption and the contact time with the aqueous media (Hansen 1983). It is well documented that roughness changes of restorative materials caused by degradation may be a factor in bacterial colonization and in the maturation of plaque on restorative materials (Quirynen & Bollen 1995).
In the pH-cycling model, a material is exposed to several pH environments alternatively for a certain time. The design was used to provide alternating demineralization (low pH) and remineralization (high pH) to model the dynamic of oral situation (Kawasaki & Featherstone 1997). The model can be considered to simulate clinical situations. The restorative materials in oral cavity are continuously exposed to pH change due to intake of foods, beverages, etc. Although the degradation of a restorative material may occur in acidic or basic solution, the pH-cycling can act on an aging process of the material. Turssi et al. (2003) reported that the pH-cycling condition had the lowest abrasion for glass ionomer and polyacid-modified composite resin compared to the artificial saliva and distilled water. However, the influence of pH-cycling on composites has not been widely studied yet.
The purpose of this study was to investigate the influence of three different storage media on chemical degradation of two composite resins by measuring changes of Knoop hardness and surface roughness and weight of the materials immersed in (a) pH-cycling condition, (b) acidic solution, and (c) distilled water. The null hypothesis was the degradation of composites subjected to a pH-cycling condition would be significantly lower than degradation in acid and distilled water.
Ⅱ. MATERIALS AND METHODS
Details of the two tested composite materials are presented in Table 1. The composites were placed in a cylindrical brass mold (10 mm in diameter, 2 mm in height) and covered with a transparent polyester film. A glass slide was placed over the film and pressure was applied to expel excess materials. Then the sample was cured separately from the surface and bottom with the standard halogen curing light for 40 s (Lee JH et al. 2014). And then the surface of the specimen were polished using 800- and 2000-grit wet abrasive papers to produce a smooth, uniform surface. The samples were rinsed with water and dried with an absorbent paper. 45 specimens were made for each composite and stored in distilled water at 37℃ for 24 h. After the samples were dried with absorbent paper, fifteen samples of each material were randomly distributed for measuring Knoop hardness, surface roughness and weight.
The initial Knoop hardness of each sample was recorded from each specimen using a Microhardness Tester (FM-7, Future-Tech Corp., Tokyo, Japan) for five times. A Knoop diamond indenter was applied under a load of 25 g for 10 s and the length of the indentation’s long diagonal measured at 400× magnification after the applied load was removed. The average of the five Knoop hardness measurements was used as the baseline value (H1) for each sample. The surface roughness of the specimens was measured using a surface measuring instrument (Surftest SV-402, Mitutoyo Corp, Kawasaki, Japan) and expressed in Ra values (μm). The tracings were carried out for five times, in five different locations, for each surface. The baseline roughness (R1) of each sample was obtained by the arithmetic mean of these readings. The initial weight was measured using a balance (AP210S; Ohaus Corp., Pine Brook, NJ, USA) to 0.0001 g accuracy. Each specimen was dried with absorbent paper to remove excess water. Then all the samples were weighted every day during a 2 weeks period, until they reached a constant weight (no more than ± 0.1 mg in any 24 h on five consecutive days of measurement). The mean of the last five measurements was considered as the initial weight (W1) for each sample.
After the initial measurement, three samples of each measurement were separately immersed in sealed glass tube filled with 20 ml following storage solutions (Keyf & Yalçin 2005) at 37℃: (a) pH-cycling condition, (b) HCl (pH 4.0 ± 0.1), and (c) distilled water. The pH values were determined by pH meter (PHM210, MeterLab, Villeurbanne Cedex, France). To reproduce the pH cycling condition, a modified protocol presented by Serra & Cury (1992) was employed for this study. The samples were first stored in HCl for 6 h and rinsed with distilled water. Then the sample was placed in the distilled water for 18 h. All of the solutions were replaced every day.
After the storage of 3 days, 1 week and 2 weeks, all of the measurements were repeated to compare the values with those of initial measurement. The changes of hardness (H), roughness (R) and weight (W) were calculated using the following equations:
H = H1 – H2, R = R1 – R2, W = W1 - W2
where H1, R1, W1 are the Knoop hardness, surface roughness, weight pre-conditioning and H2, R2, W2 are the values of three tests after conditioning.
In addition, after two weeks, the surface of one randomly selected specimen from each medium of each material was analyzed with a field emission-scanning electron microscope (FE-SEM, JSM-6700F, JEOL, Tokyo, Japan) after gold coating.
All of the data were submitted to analysis of variation (ANOVA) between different storage time and media. The significance level was determined at P = 0.05.
Ⅲ. RESULTS
Tables 2-4 present the mean H, R, and W values of EX and AP at different aging time. Negative values, which indicate an increase in hardness, roughness and weight, was observed. The hardness was decreased with the storage time in all media except AP conditioned in pH-cycling and water for 3 days. There was a significant difference in HK values (P < 0.05) over 1 week.
Regarding roughness, the two materials became rougher with exception of two cases: AP immersed pH-cycling and EX immersion in water for 3 days. After the analysis of roughness values using ANOVA, it is referred that EX was increased significantly (P < 0.05), but AP had no significant difference (P > 0.05) after 2 weeks.
During two weeks, the weights of the two materials nearly had no change. There were no significant differences in W among the storage time. However, the weight of EX was decreased, whereas AP was increased except that the sample immersed in acid. Even though there were no significant difference in H, R, and W between media for the two materials, pH-cycling and water demonstrated lower wear compared with acid solution.
The SEM macrographs of the two materials after exposure for two weeks are shown in Figures 1 and 2. There was no apparent loss of fillers or topography alterations after aging in the different media. However, the lost of matrix was minimal after immersed in pH-cycling and water when compared with acid.

SEM images of EX surface: (a) before aging (b) immersed in pH-cycling model for 2 weeks (c) immersed in the HCl for 2 weeks (d) immersed in the distilled water for 2 weeks.
Ⅳ. DISCUSSION
The chemical environment is an important aspect of the oral environment that could have an appreciable influence on restorative materials (Lee W, 2014). In this study, the three basic chemical conditions―pH-cycling, HCl and distilled water, were used. To minimize the effect of the oxygen-inhibited layer, which may influence the results, the composites were cured against a polyester transparent film.
Hardness may be defined as the resistance to permanent indentation or penetration. However, it is difficult to formulate a definition that is completely accepted, as any test method will involve a complex interaction of stresses in the test material from the force applied. Despite this condition, the most common concept of hard and soft substances is the relative resistance to penetration when immersed in chemical solution (Yap et al. 2001). The solvent molecular penetrates into the network of the resin matrix and the matrix will swell. This results in increase of the distance between the polymer chains and produces weaker polar interactions between the separated chains, which results in a softer and more wear-susceptible matrix.
In this study, the hardness of the two composite resins decreased significantly after two weeks when immersed in the three different conditions (Kim GR et al. 2014). The present study agreed with the results of others studies: decreased in Knoop hardness was found in the commercially available composites stored in water for 30 days (Watts et al. 1986); Helvatjoglou-Antoniadi et al. (1991) assumed that the changes in superficial hardness were due to the plasticization by water in the material. The results of the present study were also consistent with the results of Ferracane et al. (1998) for the unaged and aged specimens. However, contrary to these findings, Chadwick et al. (1990) found that composite resin stored in water did not significantly influence surface hardness during the 1 year study period.
There have been studies indicating that the structure of composite resin is disintegrated at low pH predominantly due to hydrolytic degradation (Chadwick et al. 1990; Geurtsen et al. 1999). As a clinical consequence, these restoratives can suffer increased wear, which has been related to the development of surface roughness leading to high friction, which sustains the rate of material detachment and may be responsible for bacterial biofilm retention and staining. The results of roughness change are shown in Table 3. The two materials were subjected to the same initial polishing treatment and presented an increase in surface roughness. However, after two weeks, AP did not present great differences regarding the roughness change when compared to EX.
The weights of the two materials used in this study nearly had no change after two weeks. But a negative weight loss, which indicated an increase in weight, was observed with AP except immersed in acid solution. When the samples were placed in the solutions, the hydrolysis of ester groups occurred on the surface of the resin matrix. However, because the monomer of resin matrix was consisting of dimethacrylate, if the matrix chain is ruptured at one side, the course of the hydrolysis of ester absorbs the water molecular. Then the weight of the matrix is increased. On the contrary, if the hydrolysis occurred at the two sides, the alcohol molecular debonds from the resin matrix. Then the weight is decreased. The course is presented in Figure 3.
In addition, when the samples are immersed in mediums containing molecules like water in this study that can diffuse into matrix, the absorbed water molecular also can improve the weight. After two weeks, the EX had more weight loss than AP. This may be because the EX contains Bis-EMA with the hydrophobic rigid aromatic nuclei and absence of the hydrophilic hydroxyl groups of Bis-GMA resin contained. It absorbed less water than Bis-GMA.
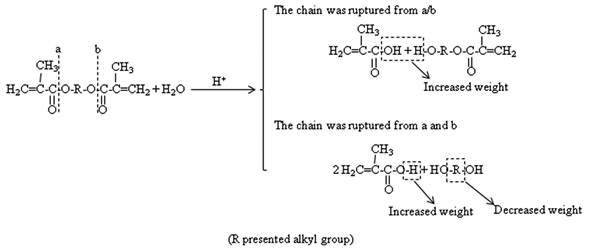
Schematic presented the hydrolysis of resin matrix. The weights of the two materials used in this study nearly had no change after two weeks. But a negative weight loss, which indicated an increase in weight, was observed with AP, except immersed in the acid solution. When the samples were placed in the solutions, the hydrolysis of ester groups occurred on the surface of the resin matrix. However, because the monomer of resin matrix was consisting of dimethacrylate, if the matrix chain is ruptured at one side, the course of the hydrolysis of ester absorbs the water molecular. Then the weight of the matrix is increased. On the contrary, if the hydrolysis occurred at the two sides, the alcohol molecular debonds from the resin matrix. Then the weight is decreased. The course is presented in Figure 3. When the chain was ruptured from the place of “a” or “b”, the weight of the matrix was increased. And when the chain was ruptured from the place both “a” and “b”, the weight of the matrix was decreased.
With regard to H, R, W of the two composite resins, there were no significant differences among storage media. However, Turssi et al. (2003) reported that for the specimens made of resin-modified glass ionomer and polyacid-modified composite resin, the pH-cycling model provided a lower surface roughness compared with water and artificial saliva. This may be attributed to the composite resin presence of a composite resin TEGDMA, a diluting monomer that can decrease the surface softening by acids (Asmussen 1984). In addition, this monomer increased the degree of polymerization and improved the physical properties of the polymer and thereby minimizing their degradation rate (Kawai et al. 1998). Although no statistical difference was detected among storage media, pH-cycling and water conditions showed the less degradation compared to acid condition according to H, R and W. It can be speculated that the changes in pH will not affect the degradation speed of restorative in oral environment. It maybe takes a long immersed time to show the significant difference for composite resin.
In addition, the SEM examination of the unconditioned surface of the two materials and specimens kept in the different media after two weeks reveals changes in surface texture. Even though there is no apparent loss of fillers or topography alterations on the surfaces of specimens after two weeks, the specimens lost less matrix after immersed in pH-cycling and water when compared with acid.
Ⅴ. CONCLUSION
Based on the results of this study, the anticipated hypotheses were rejected. The two materials showed statistically significant Knoop hardness increase after two weeks. EX presented rougher than AP after aging for two weeks. However, the weights of the two materials nearly have no change even after two weeks.
Ⅵ. ACKNOWLEDGEMENTS
This research was supported by Basic Science Research Program through the National Research Foundation of Korea (NRF) funded by the Ministry of Education (2013R1A1A2061732).
Ⅶ. REFERENCES
- Asmussen, , (1984), Softening of BISGMA-based polymers by ethanol and by organic acids of plaque, Scand J Dent Res, 92, p257-261.
-
Chadwick, RG., McCabe, JF., Walls, AW., Storer, R., (1990), The effect of storage media upon the surface microhardness and abrasion resistance of three composites, Dent Mater, 6, p123-128.
[https://doi.org/10.1016/S0109-5641(05)80042-9]
-
Ferracane, JL., (2006), Hygroscopic and hydrolytic effects in dental polymer networks, Dent Mater, 22, p211-222.
[https://doi.org/10.1016/j.dental.2005.05.005]
-
Ferracane, JL., Berge, HX., Condon, JR., (1998), In vitro aging of dental composites in water--effect of degree of conversion, filler volume, and filler/matrix coupling, coupling. J Biomed Mater Res, 42, p465-472.
[https://doi.org/10.1002/(SICI)1097-4636(19981205)42:3<465::AID-JBM17>3.0.CO;2-F]
-
Geurtsen, W., Leyhausen, G., Garcia-Godoy, F., (1999), Effect of storage media on the fluoride release and surface microhardness of four polyacid-modified composite resins (“compomers”), Dent Mater, 15, p196-201.
[https://doi.org/10.1016/S0109-5641(99)00034-2]
-
Hansen, EK., (1983), After-polymerization of visible light activated resins: surface hardness vs. light source, Scand J Dent Res, 91, p406-410.
[https://doi.org/10.1111/j.1600-0722.1983.tb00837.x]
-
Helvatjoglou-Antoniadi, M., Papadogianis, Y., Koliniotou-Kubia, E., Kubias, S., (1991), Surface hardness of light-cured and self-cured composite resins, J Prosthet Dent, 65, p215-220.
[https://doi.org/10.1016/0022-3913(91)90164-R]
-
Kawai, K., Iwami, Y., Ebisu, S., (1998), Effect of resin monomer composition on toothbrush wear resistance, J Oral Rehabil, 25, p264-268.
[https://doi.org/10.1111/j.1365-2842.1998.00246.x]
-
Kawasaki, K., Featherstone, JD., (1997), Effects of collagenase on root demineralization, J Dent Res, 76, p588-595.
[https://doi.org/10.1177/00220345970760011001]
- Keyf, F., Yalçin, F., (2005), The weight change of various light-cured restorative materials stored in water, J Contemp Dent Pract, 6, p72-79.
-
Prakki, A., Cilli, R., Mondelli, RF., Kalachandra, S., Pereira, JC., (2005), Influence of pH environment on polymer based dental material properties, J Dent, 33, p91-98.
[https://doi.org/10.1016/j.jdent.2004.08.004]
-
Quirynen, M., Bollen, CM., (1995), The influence of surface roughness and surface-free energy on supra- and subgingival plaque formation in man. A review of the literature, J Clin Periodontol, 22, p1-14.
[https://doi.org/10.1111/j.1600-051X.1995.tb01765.x]
-
Roulet, JF., Walti, C., (1984), Influence of oral fluid on composite resin and glass-ionomer cement, J Prosthet Dent, 52, p182-189.
[https://doi.org/10.1016/0022-3913(84)90092-1]
-
Sarkar, NK., (2000), Internal corrosion in dental composite wear, J Biomed Mater Res, 53, p371-380.
[https://doi.org/10.1002/1097-4636(2000)53:4<371::AID-JBM11>3.0.CO;2-N]
- Serra, MC., Cury, JA., (1992), The in vitro effect of glass-ionomer cement restoration on enamel subjected to a demineralization and remineralization model, Quintessence Int, 23, p143-147.
-
Thomas, TR., (1998), Trends in surface roughness, International Journal of Machine Tools and Manufacture (Int J Mach Tools Manuf), 38, p405-411.
[https://doi.org/10.1016/S0890-6955(97)00084-9]
-
Turssi, CP., Hara, AT., de Magalhaes, CS., Serra, MC., Rodrigues, AL. Jr., (2003), Influence of storage regime prior to abrasion on surface topography of restorative materials, J Biomed Mater Res B Appl Biomater, 65, p227-232.
[https://doi.org/10.1002/jbm.b.10005]
-
van Groeningen, G., Jongebloed, W., Arends, J., (1986), Composite degradation in vivo, Dent Mater, 2, p225-227.
[https://doi.org/10.1016/S0109-5641(86)80018-5]
-
Watts, DC., Amer, OM., Combe, EC., (1987), Surface hardness development in light-cured composites, Dent Mater, 3, p265-269.
[https://doi.org/10.1016/S0109-5641(87)80085-4]
-
Watts, DC., McNaughton, V., Grant, AA., (1986), The development of surface hardness in visible light-cured posterior composites, J Dent, 14, p169-174.
[https://doi.org/10.1016/0300-5712(86)90020-5]
-
Wu, W., McKinney, JE., (1982), Influence of chemicals on wear of dental composites, J Dent Res, 61, p1180-1183.
[https://doi.org/10.1177/00220345820610101501]
-
Yap, AU., Tan, SH., Wee, SS., Lee, CW., Lim, EL., Zeng, KY., (2001), Chemical degradation of composite restoratives, J Oral Rehabil, 28, p1015-1021.
[https://doi.org/10.1046/j.1365-2842.2001.00760.x]
-
김규리, 김동애, 김남석, 양태현, 이해형, (2014), 압입 하중과 침적 기간에 따른 의치상용 레진의 비커스 경도, 대한치과재료학회지, 41, p155-160.
[https://doi.org/10.14815/kjdm.2014.41.3.155]
-
이욱, 정재은, 정경화, 손성애, 허복, 권용훈, 박정길, (2014), 레진 색과 착색음료에 따른 나노입자형 복합레진의 반투명도와 색 변화, 대한치과재료학회지, 41, p121-128.
[https://doi.org/10.14815/kjdm.2014.41.2.121]
-
이주희, 노정훈, 설효정, 김형일, 권용훈, (2014), 각기 다르게 분류되는 methacrylate 기반의 복합레진 중합 평가, 대한치과재료학회지, 41, p273-279.
[https://doi.org/10.14815/kjdm.2014.41.4.273]