
Biomaterial-Based Tissue Engineering for Tooth-Supportive Complex Regeneration


초록
조직공학은 질병이나 사고등으로 인해 손상된 조직을 대체하는 인공 보철물 개발에서 자기 조직으로의 재생과 성장을 통해, 본래의 생리학적 기능 회복을 이끄는 재생의학으로 발전해왔다. 줄기세포등을 주입하여 재생을 이끄는 기술을 비롯해, 다양한 단백질과 성장인자들을 전달하여 손상 조직이나 장기를 복원하는 기술들이 개발되면서 조직공학 및 재생의학은 새로운 패러다임을 만들어가고 있다. 특히, 치아를 지지하는 치주조직의 재생은 수백 마이크론단위의 공간내에 특정 방향성을 지닌 섬유결합조직이 골조직과 융합하여 치아를 단단히 지지하는 복합구조를 지니고 있어, 기존의 재생술식으로는 한계가 있다. 이를 극복하고 백악질-치주인대-골조직으로 구성된 치주복합조직의 재생을 유도하기위해, 천연 및 합성 생체고분자재료를 기반으로한 다양한 의공학적 접근법들이 최근 소개되고 있다. 본고에서는 생체재료가 지니는 생물학적 특성을 기반한 치주조직재생유도 구조체 개발의 최신 연구개발 현황 및 전망을 기술하였다.
Keywords:
Biomaterials, Dental tissue engineering, Regenerative medicine, BiopolymersⅠ. INTRODUCTION
1. Tissue Engineering and Regenerative medicine
Tissue engineering and regenerative medicine are rapidly emerging in the medical, dental and engineering fields as a viable tool for regenerative medicine applications. Currently, various studies are progressing that seek to regenerate lost tissues as a consequence of disease destruction by using developed biocompatible and biofabricable materials, containing stem cells or bioactive signaling molecules (Fig. 1) (Somerman et al., 1999; Wang et al., 2005). However, new tissue formation for hierarchical multiple tissue structures is still unpredictable and challenging for regenerations of biologically-responsible and physiologically-restorable complex structures (Park et al., 2012). In order to improve tissue restoration rate, bioactive molecules (including growth factors or proteins) have been actively considered for the stimulation of tissue formation or the optimization of tissue maturation in preclinical and clinical scenarios (Chen et al., 2011; Jin et al., 2004; Kaigler et al., 2006; Singh and Suresh, 2012). In addition to bioactive molecules, bioengineered microenvironments or scaffolds have been focused on the key element to guide tissue regenerations (Taba et al., 2005). Of various transplantable materials, biodegradable materials for scaffolding systems are widely applied to control biomolecule-releasing mechanisms with local delivery systems, guide target tissue regenerations as spatiotemporal platforms, or provide optimal space for new tissue growth and maturation in diseased defects or wound sites (Bartold et al., 2016; Ivanovski et al., 2014; Mao et al., 2006; Moioli et al., 2007).
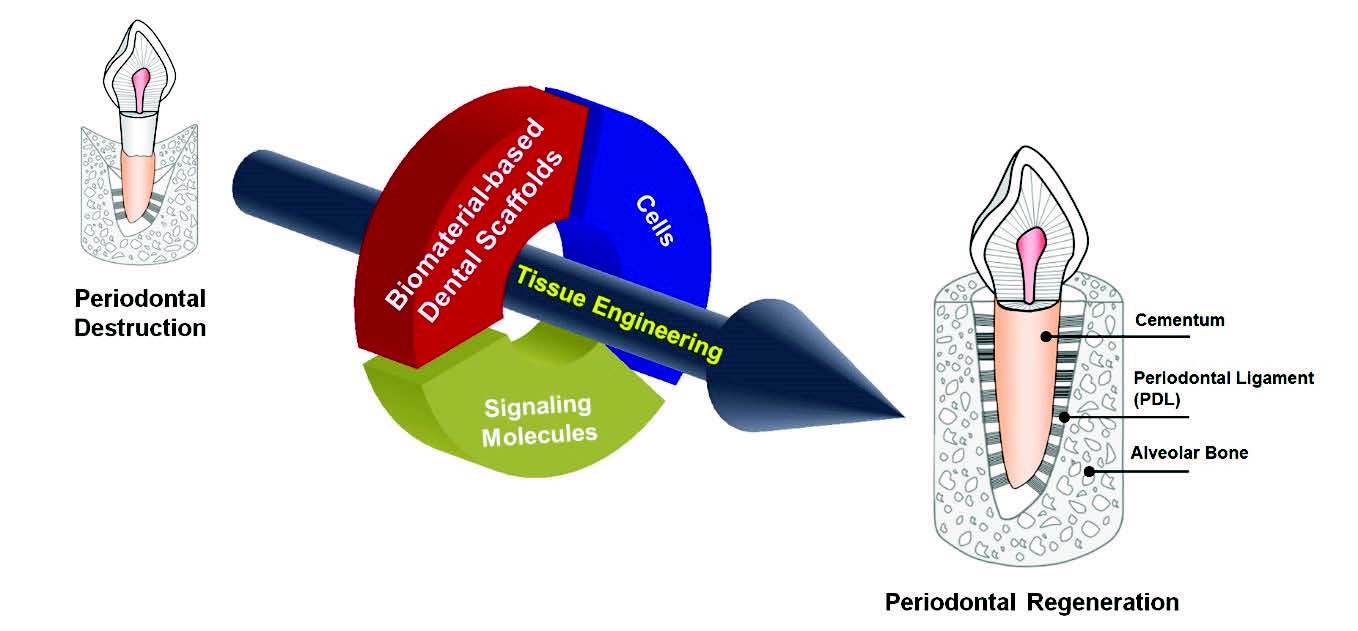
The schematic illustration for the tissue engineering strategy in periodontal tissue regenerations. Biomaterial-based scaffold, signaling molecules, and various cell types contribute to guide tissue formations and functioning restorations to support tooth structures.
As revolutionary paradigm shifts from tissue replacement to tissue regeneration, scaffold designing and manufacturing have rapidly developed in order to provide appropriate biological and engineered microenvironments for tissue regenerations (Chan and Leong, 2008). In particular, for complex-heterogenic dental tissue formation, multi-layered constructs by polymer/polymer or polymer/ceramic materials are utilized for periodontal complexes or multiple tissue repairs(Jin et al., 2008; Schek et al., 2006; Wei and Ma, 2004; Wei et al., 2007).
Of many different dental tissue regeneration approaches, the periodontal tissue engineering is challenging due to micro-scaled hierarchical tissue constructs, soft-/hard-tissue interfaces, and tissue integrations for tooth-supportive functioning restorations (Ma, 2004). In the aspect of biopolymeric material applications, there are recent advancements using natural polymeric materials; artificial extracellular matrix (ECM) with nano-fibrous morphologies (Holzwarth and Ma, 2011; Ma, 2008; Song et al., 2012) and fibrous tissue-guiding scaffolds with nano-/micro-longitudinal pores (Park et al., 2014a).
2. Natural Biopolymeric material Applications for Periodontal Tissue Regeneration
Gelatin material (or denatured collagen matrix) is generally utilized for periodontal tissue regeneration in preclinical and clinical situations (Chen and Ma, 2004; Ma, 2004). Although the collagen material have been widely utilized for preclinical and clinical regeneration treatments, it has the immune responses, uncontrollable physical properties, or unpredictable pathogen transmission rather than gelatin. Gelatin has good engineering properties like easy fabrication and limitedly controllable mechanical properties in the cross-linking process (Kamaly et al., 2016; Liu et al., 2009). Therefore, many advanced tissue engineering strategies significantly consider gelatin materials to manufacture highly porous scaffolds for tissue repair and regeneration (Ma, 2004). Nano-fibrous constructs based on natural polymeric materials have been manufactured using phase separation or peptide self-assembly methods (Beachley and Wen, 2010; Ma, 2004). For this approach, natural biopolymers like gelatin or collagen, which is FDA-approved are characterized to use as natural tissue platforms and has bioactive components like ECM compositions (Qi et al., 2015; Zhu and Marchant, 2011). In structural, nano-fibrous morphologies facilitate to promote cell activities, tissue neogeneses, or maturation of regenerated tissues (Wang et al., 2013).
In periodontal complex surrounding tooth structures, specific orientations of periodontal ligament (PDL) play a critical role to support tooth structures under mastication and occlusion microenvironments. Due to micron-scaled interfaces around tooth surface, oriented PDL regeneration has been challenging with traditional manners like randomly porous architecture transplantations or membrane placements (Park et al., 2014b; Park et al., 2016). Recently, Park et al. investigated spatiotemporal geometries in gelatin architectures using the freeze-casting method (Park et al., 2014a). To mimic the oblique PDL interface, which is approximately 70-80% of four different classified PDL interfaces, this study attempted to control the directions of ice crystal formations (Park et al., 2014a). Therefore, the directional freeze-casting of gelatin materials facilitate to have structural similarity to natural oblique PDL architectures (Park et al., 2014a).
3. Biomaterials to Develop Engineered Microenvironments for Dental Tissues: Scaffolding Systems
Of various tissue regeneration strategies, the scaffolding system plays a crucial role in defining the 3-D geometry and biomimetic microenvironment for cells and tissues (Lutolf and Hubbell, 2005; Ma, 2004; Messenger and Tomlins, 2011). A variety of materials for the developments of implantable devices have been explored for mineralized tissue regeneration like bone or teeth (Moffat et al., 2008; Spalazzi et al., 2008). Although certain metallic materials are excellent choice for medical implants for mechanical properties (Catledge et al., 2004), the main drawback as scaffold materials is non-biodegradable properties in physiological environments for regenerative medicine (Liu and Ma, 2004). The inorganic or ceramic materials like calcium phosphate (CaP)-based materials are widely applied for bone tissue regeneration due to the high osteoconductivity such as tooth-extraction socket healing as socket filling materials (De Coster et al., 2011), alveolar bone regeneration after periodontal treatments (Isidor et al., 1985; Polimeni et al., 2006), or bone ridge augmentation for dental implant stabilities (Handelsman, 2006; Kang et al., 2015). However, they have brittle properties or unpredictable spatial shrinkage in the procedures of sintering materials for manufacturing porous architectures at high temperature (Shahrjerdi et al., 2011).
For regenerative approaches instead of replacement of damaged tissues, synthetic polymer-based biomaterials with biocompatibility and biodegradability have great design potential to chemically or physically fabricate for the specific required characterizations (Liu et al., 2012; Ma, 2004). In particular, poly(lactic acid) (or polylactide; PLA), poly(glycolic acid) (or polyglycolide; PGA), and their copolymers (PLGA) have been mainly fabricated to manufacture biodegradable scaffolds with the Food and Drug Administration (FDA) approvals due to the rapid biodegradability (Liu et al., 2012; Ma, 2004; Wei and Ma, 2008; 2009). During degradation procedure, the products of PGA, PLA and PLGA by hydrolysis or enzymatic degradation are nontoxic, natural metabolites, and completely formed as carbon dioxide andwater. Using highly porous spatiotemporal scaffolds by biopolymeric materials, the dental tissues like enamel, dentin, and pulp tissues have been regenerated in various preclinical scenarios (Horst et al., 2012; Sharma et al., 2014). Moreover, drug-loaded biopolymeric scaffold strategies have the significant potential to control release rates of gene therapy vectors, proteins, or growth factors as well as provide spatiotemporal microenvironments for tissue growth and cell activities like differentiation, proliferation, or migration (Vo et al., 2012).Therefore, the biopolymeric materials are actively utilized for tissue engineering and regenerative medicine. However, the major challenge in the dental tissue engineering is recently the strategic developments of micron-scaled complex geometries to guide optimal tissue complexes using biodegradable polymeric materials (Albuquerque et al., 2014; Park et al., 2014b).Therefore, customized or flexible approaches using biopolymers are significantly required for complex geometries or non- standardized, unpredictable defects inthe periodontal tissue engineering.
4. Advanced Strategies for Tooth-Supportive Tissue Regenerations
The periodontium is composed of four specific tissues: gingiva (fibrous tissue), cementum (mineralized layer on the root surface with 150-200 μm thickness), PDL (250-300 μm thick space with perivasculature and Sharpey’s fibers), and alveolar bone (mineralized tissue to support tooth structures) (Park et al., 2014a). The PDL, a fibrous connective tissue bundle is oriented perpendicular/oblique to the tooth root surface with anchorage between the bone surface and cementum layer (Park et al., 2012; Park et al., 2014b) and provide mechanical anchorage to support occlusion/ mastication loading (Park et al., 2014a). However, periodontal disease, which is a common and highly prevalent inflammatory infectious disease affecting periodontia leads to destruction of the periodontal complex involving alveolar bone-PDL interface degeneration and subsequent tooth loss (Park et al., 2012).
To regenerate periodontal complex from diseaseassociated destructions, fiber-guiding scaffoldswere currently developed with the architectural compartmentalization for periodontal complexes composed with PDL, alveolar bone, and cementum (Park et al., 2010; Park et al., 2012; Park et al., 2014b). The 3-D reconstructed images after CT-scanning the periodontal fenestration defects, the wax molds for multi-layered scaffolds were designed and manufactured by the computer design tools and the 3-D wax printing system (Park et al., 2012; Park et al., 2014b). After casting the biopolymeric material (PCL), customized PCL scaffolds were transplanted to promote tooth-suportive structures; bone formation, PDL regeneration with perpendicular/oblique orientations to the tooth root surface, and cementum deposition on the root surface (Park et al., 2012; Park et al., 2014b). Interestingly, the fibrous connective tissues were integrated with the mineralized tissue and the restoration of tissue functioning was identified using the periostin staining method (Park et al., 2012).
In addition to 3-D printing technology the cell sheet technology is one promising approach for clinical applications in periodontal tissue regeneration (Bartold et al., 2016; Iwata et al., 2010; Iwata et al., 2015). After cultivation of PDL cells on chemically-modified cell culture plates for a single sheet, multiple PDL cell sheets were stacked using woven PGA membrane and transplanted to periodontal defects with β-tricalcium phosphate (β–TCP), which is an osteoconductive material for bone regeneration (Iwata et al., 2009; Washio et al., 2010). Particularly, PGA having high hydrophilicity facilitates to inhibit shrinkage of a mono-layered cell construct (Iwata et al., 2009), easily and rapidly stack multiple cell sheets (Iwata et al., 2009; Washio et al., 2010), and adjust to any defect geometries with the high flexibility like a paper (Ishikawa et al., 2009). Moreover, rapid biodegradability of woven PGA constructs can promote the tissue integration with mineralized tissues, which β–TCP with osteogenic characterization contributed to regenerate (Tsumanuma et al., 2011; Washio et al., 2010). As results demonstrated, multi-layered PDL cell with an osteoconductive material (β–TCP) promote to orient PDL fibrous tissues with tissue integration on cementum and bone surfaces in multi-compartmentalized periodontia (Iwata et al., 2009; Tsumanuma et al., 2011).
The biphasic scaffold with PDL cell sheets was developed using electrospun nanofibrous PCL membrane which was for immobilization and positional transplantation of PDL on the periodontal defect sites (Ivanovski et al., 2014; Vaquette et al., 2012). Moreover, this highly porous PCL membrane can be easily fabricated to improve osteoconductivity after calcium phosphate (CaP) deposition on surface and facilitated osteogeneic acceleration to regenerate tooth-supportive mineralized tissues (Dan et al., 2014; Obregon et al., 2015; Vaquette et al., 2013).
Ⅱ. CONCLUSIONS
Tissue engineering and regenerative medicine is one of promising interdisciplinary strategies to introduce new tissue neogeneses around diseased or injured destructions and improve tissue functions. There are three components in tissue engineering such as signaling biomolecules, bioactive cell types, and biomaterial-based scaffolds. In particular, complicated geometries or systematic tissue integrations should be considered highly biocompatible platforms to guide and grow cells and tissues with customized architectures. Therefore, as we have demonstrated in this review, the developments of novel biomaterials-based tissue engineering techniqueshave been crucially required and clinical reconstructions should be considered for periodontal complex regeneration due to the physiologically complicated and difficult accessible topographies. As Rasperini et al. recently reported the first clinical approach using the 3-D printed scaffold for periodontal regeneration (Rasperini et al., 2015), we still need to explore and investigate the optimal and ideal biomaterials to have better characteristics for specific target tissues in periodontal and dental applications.
Acknowledgments
This work was supported by the National Research Foundation of Korea (NRF-2014R1A1A2059301).
DISCLOSURE
The author has no conflict of interest for this work.
References
-
Albuquerque, MT., Valera, MC., Nakashima, M., Nor, JE., Bottino, MC., (2014), Tissue-engineering-based strategies for regenerative endodontics, J Dent Res, 93(12), p1222-1231.
[https://doi.org/10.1177/0022034514549809]
-
Bartold, PM., Gronthos, S., Ivanovski, S., Fisher, A., Hutmacher, DW., (2016), Tissue engineered periodontal products, J Periodontal Res, 51, p1-15.
[https://doi.org/10.1111/jre.12275]
-
Beachley, V., Wen, X., (2010), Polymer nanofibrous structures: Fabrication, biofunctionalization, and cell interactions, Prog Polym Sci, 35, p868-892.
[https://doi.org/10.1016/j.progpolymsci.2010.03.003]
-
Catledge, SA., Vohra, YK., Bellis, SL., Sawyer, AA., (2004), Mesenchymal stem cell adhesion and spreading on nanostructured biomaterials, J Nanosci Nanotechno, l4, p986-989.
[https://doi.org/10.1166/jnn.2004.137]
-
Chan, BP., Leong, KW., (2008), Scaffolding in tissue engineering: general approaches and tissue-specific considerations, Eur Spine J 17 Suppl, 4, p467-479.
[https://doi.org/10.1007/s00586-008-0745-3]
-
Chen, FM., An, Y., Zhang, R., Zhang, M., (2011), New insights into and novel applications of release technology for periodontal reconstructive therapies, J Control Release, 149, p92-110.
[https://doi.org/10.1016/j.jconrel.2010.10.021]
-
Chen, VJ., Ma, PX., (2004), Nano-fibrous poly(L-lactic acid) scaffolds with interconnected spherical macropores, Biomaterials, 25, p2065-2073.
[https://doi.org/10.1016/j.biomaterials.2003.08.058]
-
Dan, H., Vaquette, C., Fisher, AG., Hamlet, SM., Xiao, Y., Hutmacher, DW., et al , (2014), The influence of cellular source on periodontal regeneration using calcium phosphate coated polycaprolactone scaffold supported cell sheets, Biomaterials, 35, p113-122.
[https://doi.org/10.1016/j.biomaterials.2013.09.074]
-
De Coster, P., Browaeys, H., De Bruyn, H., (2011), Healing of extraction sockets filled with BoneCeramic(R) prior to implant placement: preliminary histological findings, Clin Implant Dent Relat Res, 13, p34-45.
[https://doi.org/10.1111/j.1708-8208.2009.00184.x]
-
Handelsman, M., (2006), Surgical guidelines for dental implant placement, Br Dent J, 201, p139-152.
[https://doi.org/10.1038/sj.bdj.4813947]
-
Holzwarth, JM., Ma, PX., (2011), Biomimetic nanofibrous scaffolds for bone tissue engineering, Biomaterials, 32, p9622-9629.
[https://doi.org/10.1016/j.biomaterials.2011.09.009]
-
Horst, OV., Chavez, MG., Jheon, AH., Desai, T., Klein, OD., (2012), Stem cell and biomaterials research in dental tissue engineering and regeneration, Dent Clin North Am, 56, p495-520.
[https://doi.org/10.1016/j.cden.2012.05.009]
-
Ishikawa, I., Iwata, T., Washio, K., Okano, T., Nagasawa, T., Iwasaki, K., et al , (2009), Cell sheet engineering and other novel cell-based approaches to periodontal regeneration, Periodontol, (2000), 51, p220-238.
[https://doi.org/10.1111/j.1600-0757.2009.00312.x]
-
Isidor, F., Attstrom, R., Karring, T., (1985), Regeneration of alveolar bone following surgical and non-surgical periodontal treatment, J Clin Periodontol, 12, p687-696.
[https://doi.org/10.1111/j.1600-051X.1985.tb00940.x]
-
Ivanovski, S., Vaquette, C., Gronthos, S., Hutmacher, DW., Bartold, PM., (2014), Multiphasic scaffolds for periodontal tissue engineering, J Dent Res, 93, p1212-1221.
[https://doi.org/10.1177/0022034514544301]
-
Iwata, T., Yamato, M., Tsuchioka, H., Takagi, R., Mukobata, S., Washio, K., et al , (2009), Periodontal regeneration with multi-layered periodontal ligament-derived cell sheets in a canine model, Biomaterials, 30, p2716-2723.
[https://doi.org/10.1016/j.biomaterials.2009.01.032]
-
Iwata, T., Yamato, M., Zhang, Z., Mukobata, S., Washio, K., Ando, T., et al , (2010), Validation of human periodontal ligament-derived cells as a reliable source for cytotherapeutic use, J Clin Periodontol, 37, p1088-1099.
[https://doi.org/10.1111/j.1600-051X.2010.01597.x]
-
Iwata, T., Washio, K., Yoshida, T., Ishikawa, I., Ando, T., Yamato, M., et al , (2015), Cell sheet engineering and its application for periodontal regeneration, J Tissue Eng Regen Med, 9, p343-356.
[https://doi.org/10.1002/term.1785]
-
Jin, Q., Anusaksathien, O., Webb, SA., Printz, MA., Giannobile, WV., (2004), Engineering of tooth-supporting structures by delivery of PDGF gene therapy vectors, Mol Ther, 9(4), p519-526.
[https://doi.org/10.1016/j.ymthe.2004.01.016]
-
Jin, Q., Wei, G., Lin, Z., Sugai, JV., Lynch, SE., Ma, PX., et al , (2008), Nanofibrous scaffolds incorporating PDGF-BB microspheres induce chemokine expression and tissue neogenesis in vivo, PLoS One, 3, pe1729.
[https://doi.org/10.1371/journal.pone.0001729]
-
Kaigler, D., Cirelli, JA., Giannobile, WV., (2006), Growth factor delivery for oral and periodontal tissue engineering, Expert Opin Drug Deliv, 3, p647-662.
[https://doi.org/10.1517/17425247.3.5.647]
-
Kamaly, N., Yameen, B., Wu, J., Farokhzad, OC., (2016), Degradable Controlled-Release Polymers and Polymeric Nanoparticles: Mechanisms of Controlling Drug Release, Chem Rev, 116, p2602-2663.
[https://doi.org/10.1021/acs.chemrev.5b00346]
-
Kang, YH., Kim, HM., Byun, JH., Kim, UK., Sung, IY., Cho, YC., et al , (2015), Stability of simultaneously placed dental implants with autologous bone grafts harvested from the iliac crest or intraoral jaw bone, BMC Oral Health, 15, p172.
[https://doi.org/10.1186/s12903-015-0156-x]
-
Liu, X., Ma, PX., (2004), Polymeric scaffolds for bone tissue engineering, Ann Biomed Eng, 32, p477-486.
[https://doi.org/10.1023/B:ABME.0000017544.36001.8e]
-
Liu, X., Smith, LA., Hu, J., Ma, PX., (2009), Biomimetic nanofibrous gelatin/apatite composite scaffolds for bone tissue engineering, Biomaterials, 30, p2252-2258.
[https://doi.org/10.1016/j.biomaterials.2008.12.068]
-
Liu, X., Holzwarth, JM., Ma, PX., (2012), Functionalized synthetic biodegradable polymer scaffolds for tissue engineering, Macromol Biosci, 12, p911-919.
[https://doi.org/10.1002/mabi.201100466]
-
Lutolf, MP., Hubbell, JA., (2005), Synthetic biomaterials as instructive extracellular microenvironments for morphogenesis in tissue engineering, Nat Biotechnol, 23, p47-55.
[https://doi.org/10.1038/nbt1055]
-
Ma, PX., (2004), Scaffolds for tissue fabrication, Materials Today, 7, p30-40.
[https://doi.org/10.1016/S1369-7021(04)00233-0]
-
Ma, PX., (2008), Biomimetic materials for tissue engineering, Adv Drug Deliv Rev, 60, p184-198.
[https://doi.org/10.1016/j.addr.2007.08.041]
-
Mao, JJ., Giannobile, WV., Helms, JA., Hollister, SJ., Krebsbach, PH., Longaker, MT., et al , (2006), Craniofacial tissue engineering by stem cells, J Dent Res, 85, p966-979.
[https://doi.org/10.1177/154405910608501101]
-
Messenger, MP., Tomlins, PE., (2011), Regenerative medicine: a snapshot of the current regulatory environment and standards, Adv Mater, 23, pH10-17.
[https://doi.org/10.1002/adma.201100254]
-
Moffat, KL., Sun, WH., Pena, PE., Chahine, NO., Doty, SB., Ateshian, GA., et al , (2008), Characterization of the structure-function relationship at the ligament-to-bone interface, Proc Natl Acad Sci U S A, 105, p7947-7952.
[https://doi.org/10.1073/pnas.0712150105]
-
Moioli, EK., Clark, PA., Xin, X., Lal, S., Mao, JJ., (2007), Matrices and scaffolds for drug delivery in dental, oral and craniofacial tissue engineering, Adv Drug Deliv Rev, 59, p308-324.
[https://doi.org/10.1016/j.addr.2007.03.019]
-
Obregon, F., Vaquette, C., Ivanovski, S., Hutmacher, DW., Bertassoni, LE., (2015), Three-Dimensional Bioprinting for Regenerative Dentistry and Craniofacial Tissue Engineering, J Dent Res, 94, p143S-152S.
[https://doi.org/10.1177/0022034515588885]
-
Park, CH., Rios, HF., Jin, Q., Bland, ME., Flanagan, CL., Hollister, SJ., et al , (2010), Biomimetic hybrid scaffolds for engineering human tooth-ligament interfaces, Biomaterials, 31, p5945-5952.
[https://doi.org/10.1016/j.biomaterials.2010.04.027]
-
Park, CH., Rios, HF., Jin, Q., Sugai, JV., Padial-Molina, M., Taut, AD., et al , (2012), Tissue engineering bone-ligament complexes using fiber-guiding scaffolds, Biomaterials, 33, p137-145.
[https://doi.org/10.1016/j.biomaterials.2011.09.057]
-
Park, CH., Kim, KH., Rios, HF., Lee, YM., Giannobile, WV., Seol, YJ., (2014a), Spatiotemporally controlled microchannels of periodontal mimic scaffolds, J Dent Res, 93, p1304-1312.
[https://doi.org/10.1177/0022034514550716]
-
Park, CH., Rios, HF., Taut, AD., Padial-Molina, M., Flanagan, CL., Pilipchuk, SP., et al , (2014b), Image-based, fiber guiding scaffolds: a platform for regenerating tissue interfaces, Tissue Eng Part C Methods, 20, p533-542.
[https://doi.org/10.1089/ten.tec.2013.0619]
-
Park, CH., Kim, KH., Lee, YM., Seol, YJ., (2016), Advanced Engineering Strategies for Periodontal Complex Regeneration, Materials, 9, p57.
[https://doi.org/10.3390/ma9010057]
-
Polimeni, G., Xiropaidis, AV., Wikesjo, UM., (2006), Biology and principles of periodontal wound healing/regeneration, Periodontol, (2000), 41, p30-47.
[https://doi.org/10.1111/j.1600-0757.2006.00157.x]
-
Qi, C., Yan, X., Huang, C., Melerzanov, A., Du, Y., (2015), Biomaterials as carrier, barrier and reactor for cell-based regenerative medicine, Protein Cell, 6, p638-653.
[https://doi.org/10.1007/s13238-015-0179-8]
-
Rasperini, G., Pilipchuk, SP., Flanagan, CL., Park, CH., Pagni, G., Hollister, SJ., et al , (2015), 3D-printed Bioresorbable Scaffold for Periodontal Repair, J Dent Res, 94, p153S-157S.
[https://doi.org/10.1177/0022034515588303]
-
Schek, RM., Wilke, EN., Hollister, SJ., Krebsbach, PH., (2006), Combined use of designed scaffolds and adenoviral gene therapy for skeletal tissue engineering, Biomaterials, 27, p1160-1166.
[https://doi.org/10.1016/j.biomaterials.2005.07.029]
- Shahrjerdi, A., Mustapha, F., Bayat, M., Sapuan, SM., Majid, DLA., (2011), Fabrication of functionally graded hydroxyapatitetitanium by applying optimal sintering procedure and powder metallurgy, Int J Phys Sci, 6, p2258-2267.
-
Sharma, S., Srivastava, D., Grover, S., Sharma, V., (2014), Biomaterials in tooth tissue engineering: a review, J Clin Diagn Res, 8, p309-315.
[https://doi.org/10.7860/jcdr/2014/7609.3937]
-
Singh, P., Suresh, DK., (2012), Clinical evaluation of GEM 21S((R)) and a collagen membrane with a coronally advanced flap as a root coverage procedure in the treatment of gingival recession defects: A comparative study, J Indian Soc Periodontol, 16, p577-583.
[https://doi.org/10.4103/0972-124X.106919]
-
Somerman, MJ., Ouyang, HJ., Berry, JE., Saygin, NE., Strayhorn, CL., D'Errico, JA., et al , (1999), Evolution of periodontal regeneration: from the roots’point of view, J Periodontal Res, 34, p420-424.
[https://doi.org/10.1111/j.1600-0765.1999.tb02276.x]
-
Song, W., Markel, DC., Wang, S., Shi, T., Mao, G., Ren, W., (2012), Electrospun polyvinyl alcohol-collagen-hydroxyapatite nanofibers: a biomimetic extracellular matrix for osteoblastic cells, Nanotechnology, 23, p115101.
[https://doi.org/10.1088/0957-4484/23/11/115101]
-
Spalazzi, JP., Dagher, E., Doty, SB., Guo, XE., Rodeo, SA., Lu, HH., (2008), In vivo evaluation of a multiphased scaffold designed for orthopaedic interface tissue engineering and soft tissue-to-bone integration, J Biomed Mater Res A, 86, p1-12.
[https://doi.org/10.1002/jbm.a.32073]
-
Taba, M. Jr., Jin, Q., Sugai, JV., Giannobile, WV., (2005), Current concepts in periodontal bioengineering, Orthod Craniofac Res, 8, p292-302.
[https://doi.org/10.1111/j.1601-6343.2005.00352.x]
-
Tsumanuma, Y., Iwata, T., Washio, K., Yoshida, T., Yamada, A., Takagi, R., et al , (2011), Comparison of different tissue-derived stem cell sheets for periodontal regeneration in a canine 1-wall defect model, Biomaterials, 32, p5819-5825.
[https://doi.org/10.1016/j.biomaterials.2011.04.071]
-
Vaquette, C., Fan, W., Xiao, Y., Hamlet, S., Hutmacher, DW., Ivanovski, S., (2012), A biphasic scaffold design combined with cell sheet technology for simultaneous regeneration of alveolar bone/periodontal ligament complex, Biomaterials, 33, p5560-5573.
[https://doi.org/10.1016/j.biomaterials.2012.04.038]
-
Vaquette, C., Ivanovski, S., Hamlet, SM., Hutmacher, DW., (2013), Effect of culture conditions and calcium phosphate coating on ectopic bone formation, Biomaterials, 34, p5538-5551.
[https://doi.org/10.1016/j.biomaterials.2013.03.088]
-
Vo, TN., Kasper, FK., Mikos, AG., (2012), Strategies for controlled delivery of growth factors and cells for bone regeneration, Adv Drug Deliv Rev, 64, p1292-1309.
[https://doi.org/10.1016/j.addr.2012.01.016]
-
Wang, HL., Greenwell, H., Fiorellini, J., Giannobile, W., Offenbacher, S., Salkin, L., et al , (2005), Periodontal regeneration, J Periodontol, 76, p1601-1622.
[https://doi.org/10.1902/jop.2005.76.9.1601]
-
Wang, X., Ding, B., Li, B., (2013), Biomimetic electrospun nanofibrous structures for tissue engineering, Materials Today, 16, p229-241.
[https://doi.org/10.1016/j.mattod.2013.06.005]
-
Washio, K., Iwata, T., Mizutani, M., Ando, T., Yamato, M., Okano, T., et al , (2010), Assessment of cell sheets derived from human periodontal ligament cells: a pre-clinical study, Cell Tissue Res, 341, p397-404.
[https://doi.org/10.1007/s00441-010-1009-1]
-
Wei, G., Ma, PX., (2004), Structure and properties of nano-hydroxyapatite/polymer composite scaffolds for bone tissue engineering, Biomaterials, 25, p4749-4757.
[https://doi.org/10.1016/j.biomaterials.2003.12.005]
-
Wei, G., Jin, Q., Giannobile, WV., Ma, PX., (2007), The enhancement of osteogenesis by nano-fibrous scaffolds incorporating rhBMP-7 nanospheres, Biomaterials, 28, p2087-2096.
[https://doi.org/10.1016/j.biomaterials.2006.12.028]
-
Wei, G., Ma, PX., (2008), Nanostructured Biomaterials for Regeneration, Adv Funct Mater, 18, p3566-3582.
[https://doi.org/10.1002/adfm.200800662]
-
Wei, G., Ma, PX., (2009), Partially nanofibrous architecture of 3D tissue engineering scaffolds, Biomaterials, 30, p6426-6434.
[https://doi.org/10.1016/j.biomaterials.2009.08.012]
-
Zhu, J., Marchant, RE., (2011), Design properties of hydrogel tissue-engineering scaffolds, Expert Rev Med Devices, 8, p607-626.
[https://doi.org/10.1586/erd.11.27]