
Effects of UV treatment on orthodontic microimplant surface after autoclaving
Abstract
The aim of this study was to investigate the effects of storage time and steam autoclaving on the accumulation of carbon content on the microimplant surfaces and the effectiveness of UV treatment in reducing the carbon contamination. The effects of storage time was tested using microimplants from four different years of manufacture across a span of eleven years. The effects of steam autoclaving and UV treatment on either increase or decrease of the carbon content were tested using two microimplant groups - the newly manufactured- and eleven year old microimplants. In addition to the surface carbon measurements, we further investigated the effect of surface carbon content on the biological properties of the microimplant, using a water contact angle test and cell activity test. Storage time showed a constant increase in the carbon contamination on the microimplants sufaces across eleven years. Among the newly manufactured microimplants there was a significant increase in carbon due to autoclaving and UV treatment significantly decontaminated the surface. Among the eleven years old microimplants, autoclaving did not effect the carbon content but UV treatment had a positive effect of the overall carbon content. UV treatment increased the superhydrophilicity, but there was no significant changes in the cell activity Increase in carbon contamination was due to storage time and steam autoclaving. However, UV treatment reduced the carbon contamination and increased superhydrophilicity of the microimplant surfaces.
초록
이 연구의 목적은, 마이크로임플란트의 보관기간과 증기고압멸균이 표면의 탄소함량에 미치는 영향과, 자외선 처리를 통해 탄소함량을 감소시킬 수 있는가를 조사하는 것이다. 보관기간의 영향을 조사하기 위해, 11년 동안 4개의 다른 시점에서 제작된 4개의 마이크로임플란트 그룹을 비교 실험하였고, 증기고압멸균과 자외선 처치의 효과를 평가하기 위해서는 2개 그룹 (새로 제작된 마이크로임플란트와 제조 후 11년 경과된 마이크로임플란트)을 사용하여 실험하였다. 물 접촉각 실험, 세포활성 검사를 사용하여 표면 탄소함량이 마이크로임플란트의 생물학적 특성에 미치는 영향도 함께 조사하였다. 실험결과, 11년의 보관기간 동안 마이크로임플란트 표면의 탄소함량은 점차 증가함을, 또한 증기고압멸균에 의해서 탄소함량이 증가됨을, 그리고 자외선 처리를 통해서 탄소함량을 유의하게 감소시킬 수 있음을 관찰하였다. 한편, 마이크로임플란트 표면의 탄소함량 감소에 따라 세포활성에는 주목할 변화가 없었으나 표면의 초친수성이 증가됨을 관찰하였다. 이 결과로부터, 마이크로임플란트의 긴 보관기간과 증기고압멸균처리는 표면의 탄소함량을 증가시키지만 자외선 처리는 마이크로임플란트 표면의 탄소함량을 감소시키고 초친수성을 증가시키므로 마이크로임플란트의 안정성 향상을 유도할 수 있을 것이라는 결론을 얻었다.
Keywords:
Orthodontic MicroImplants, Hydrocarbon, UV treatment키워드:
교정용 마이크로임플란트, 탄화수소, 자외선 처치Ⅰ. INTRODUCTION
Biocompatibility of titanium implants can be attributed to the presence of an oxide (TiO2) layer that forms spontaneously on the implant surfaces upon exposure to oxygen. As this layer is highly corrosion resistant and thermodynamically stable, it plays an important role in protecting the implant surface from undergoing ionization, and thereby, aides in the process of bone to implant integration (Sul YT et al. 2002). This oxide layer, however, is prone to contamination of impurity elements, which can adversely affect the biocompatibility of the implant. A variety of contaminants have been indentified on the implant surface which include calcium, chlorine, sulphur, phosphorus, silica and sodium, among which carbon was dominant (Chin MYH et al. 2007, Lausmaa J et al. 1990).
Carbon contamination of the oxide layer on the implant surface can occur in three stages. Firstly, carbon can diffuse into the oxide layer during the production processes of implant such as machine cutting, etching, cleaning and other post treatments (Buser D et al. 2004, Att W et al. 2009). The oxide layer of implant surfaces can also absorb carbon from air during the storage period. Depending on the contact time with air, the level of carbon absorption could increase. The contamination of the oxide layer by hydrocarbons takes only few seconds, initially the bonds are unsaturated, which eventually saturate over time (Haibin L et al. 2013, Olefjord I et al. 1993, Mouhyi J et al. 1998). Another contributing factor is the autoclaving process which is a must procedure for the sterilization of the implants before clinical use. Although deionized water is used for autoclaving, the possibility of carbon contamination cannot be ruled out (Serro AP et al. 2003). As the carbon contamination can reduce the bioactivity of the implant surface (Hirota M et al. 2014), resulting in ‘biological aging’ of the implant surfaces (Att W et al. 2012), and hinder the integration of implant with bone, this issue has become important in implant dentistry.
Among the widely used technological advancements, surface treatment of dental implants in on the rise (Byoung-kook kim et al., 2015). Recently, ultraviolet (UV) treatment has earned attention as a method to reduce the carbon content on the implant surface. This relatively new method was proven to be effective in decomposing the carbon contaminants absorbed and accumulated in the oxide layer, and thus, improving the physiochemical properties of the implant surface. Significant increase in the biological capability was shown to be obtainable (Tabuchi M et al. 2015). These findings obtained from dental implants and other titanium parts suggested that the UV treatment method may be used to improve the anchoring capability of the titanium orthodontic microimplants. Unfortunately, however, most of the previous studies on UV treatment focused on dental implants. Given the differences between dental and orthodontic implants in terms of material (pure titanium vs. titanium alloy) and surface texture (roughened surface vs. machined surface), the results obtained from dental implants cannot be extended directly to the orthodontic microimplants. Therefore, it is yet to be determined how effective as a procedure UV treatment is over orthodontic microimplants.
The aim of this study was to investigate 1) the effect of storage time and steam autoclaving on the accumulation of carbon content on the microimplant surfaces, and 2) the effectiveness of UV treatment in reducing the carbon content caused by prolonged storage time and/or autoclaving.
Ⅱ. MATERIALS AND METHODS
Three experiments were performed, which were respectively aimed to investigate the effects of storage time, autoclaving and UV treatment on either the increase or decrease of carbon content on the microimplant surface. Microimplants of four different manufacturing years (and as such different storage time) were used in these tests, which included those newly manufactured, and those produced six years ago, nine years ago, and eleven years ago. All the microimplants were of the Absoanchor system (SH1615-06, Dentos Inc, Daegu, Korea), which had been stored in individual packages (requiring sterilization prior to clinical usage).
1. Effect of storage time
A total of 120 microimplants - 30 from each of the four microimplant groups (newly manufactured, six years old, nine years old, eleven years old) were used in this test. The carbon content on the microimplant surfaces was measured with an X-ray photoelectron spectroscope (XPS, Thermo scientific ESCALAB 250Xi) using a monochromatized AL-Kα X-ray (1486.6 eV, 180 W) as the excitation source. The XPS scans were performed on random spots on the body of the microimplants.
2. Effect of steam autoclaving
Two microimplant groups - the newly manufactured- and eleven years old microimplants - were selected to test the effect of autoclaving. These two groups were selected because they exhibited either the lowest- or the highest carbon contents on the surfaces among the four microimplant groups in the above test, and as such that autoclaving them would lead to most marked differences. Steam autoclaving was performed under standard procedures used in the clinic, for a total of 60 microimplants (30 from each of the two microimplant groups). After the autoclaving, the carbon content on the microimplant surfaces was measured following the same procedure as described above.
3. Effect of UV treatment on decreasing the carbon content
Two microimplant groups - the newly manufactured- and eleven years old microimplants after autoclaving received UV treatment. The UV treatment was performed using a 5x8W – 254 nm tube bactericidal lamp (Bio-Link BLX- 254 Vilber Lourmat co. Suebia, Germany) for 12 minutes, based on a previous study by Hirota Metal [ 10 ]. A fter which, the carbon content on the microimplant surfaces was measured.
4. UV effect on the biological properties
In order to further investigate the effect of UV treatment in improving the biological properties of the microimplant surface, we evaluated the hydrophilicity and the activity of osteoblastic cells on microimplant surfaces. Water contact angle method was used for the evaluation of hydrophilicity. A total of 20 newly manufactured microimplants were autoclaved first and UV treatment was applied to 10 of them. Then, placing a water droplet on the microimplants, photographs were taken to measure the contact angles. We evaluated the biological properties of the microimplant materials, before and after the UV treatment, by measuring the growth of osteoblast cells thereon. Note that, because this test required a test sample larger than the microimplant diameter (1.6 mm), we fabricated testing samples from raw titanium bar (Φ 4mm). Cutting a total of 18 titanium samples (Φ 4mm x 1mm), we autoclaved them first. Nine samples received the UV treatment before being placed in the well plates. The osteoblast cells were differentiated over them, followed by a RNA isolation and quantitative real-time PCR [13]. Calvarial osteoblasts isolated from B6 neonates were cultured, incubated, stained and observed under the stereo-zoom microscope (Leica, Model S6D). Then, gene expression procedure to evaluate the affinity of osteoblast cells (RNA isolation and quantitative real-time-PCR) was done.
Statistical calculations were carried out with IBM SPSS software V22.0 for Mac OS. For the effect of storage time, one-way ANOVA and post hoc multiple comparison test of Tukey (HSD) were used for intergroup and subgroup comparison respectively. For the effect of steam autoclaving and UV treatment, and the contact angle test the independent-samples t-test was used. The results were evaluated at the significance level of p < 0.05, with a 95% confidence interval.
Ⅲ. RESULTS
1. Effect of storage time
The carbon percentage measured on the four microimplant groups with different manufacturing years are tabulated in Table 1, which clearly indicates that the older the microimplant the higher the carbon content on the surface. There is a steady increase in the percentage of 122 carbon with newly manufactured microimplants showing the lowest value of 50.86%, six years old showing 54.90%, nine years old showing 59.68% and eleven years old showing the highest value 66.87%. The ANOVA showed that all these differences were statistically significant (p<.05). Post HOC analysis showed that there was a significant difference within all the groups (p<.05).
2. Effect of autoclaving and UV treatment on the carbon content on the microimplant surface
Figure 1 compares the carbon percentage measured on the two microimplant groups (the newly manufactured-microimplants and the eleven years old microimplants). The results clearly show that autoclaving increased the carbon content whereas the UV treatment decreased the carbon content in both the newly produced group and the eleven years old microimplant group. The subgroup ‘untreated’ indicates the as-received microimplant samples, i.e., prior to autoclaving. In the case of the newly manufactured microimplant group, the untreated samples had a mean carbon percentage of 50.85%, autoclaved samples 53.78% and autoclaved + UV 48.51%. There was a statistically significant difference between the untreated and autoclaved, and autoclaved and autoclaved + UV groups. On the other hand, untreated microimplants and the autoclaved + UV group did not show a significant difference. The positive effect of UV treatment was more clearly observed in the results obtained from the eleven years old microimplants. Comparing the mean carbon content of 66.86% on the untreated microimplants, the percentages obtained from the autoclaved samples showed 67.77% and autoclaved + UV showed 53.04% as shown in Figure 1. On statistical comparison of the microimplants among the untreated and autoclaved + UV samples, there was a significant difference in the carbon percentages with a p<.05, among the samples of autoclaved and autoclaved + UV there was a statistical difference in the carbon percentages with a p<.05. On the other hand, untreated and the autoclaved samples did not show a significant difference with a p>.05.
3. UV effect on the Biological properties
Table 2 compares the results of the water contact angle test, among the autoclaved samples the mean contact angle was 74°, and that of the autoclaved + UV treated samples was 10°. Figure 2 shows the typical results. Figure 3 shows the results of the cell culture, osteoblast differentiation, and RNA isolation and quantitive real-time- PCR. Figure 3a and 3b show typical results obtained from the Autoclaved and Autoclaved + UV groups respectively, which show scattered growth of the osteoblast cells over the titanium samples. Figure 3c and 3d is a graphical representation of the RNA isolation and quantitative real-time PCR of the autoclaved and autoclaved + UV groups, which indicate that the UV treatment did not show any difference in cell growth over the titanium samples.
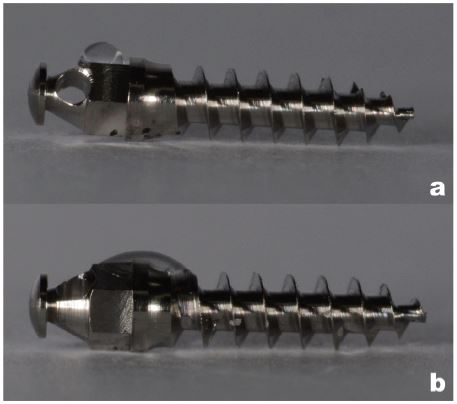
Water contact angle test results conducted on the newly manufactured microimplants; a: Autoclaved microimplants, b: Autoclaved + UV treated samples.
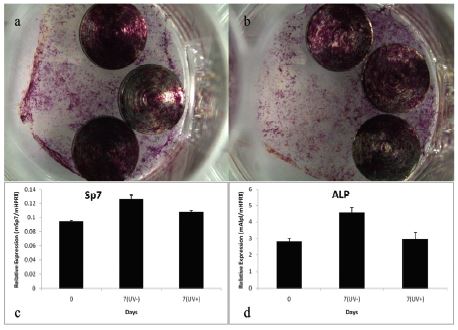
Osteoblast differentiation; a: Osteoblast differentiation in Autoclaved samples, b: Osteoblast differentiation in Autoclaved + UV treatment samples. RNA isolation and quantitative real-time-PCR; c: Results showing the relative expression of mSp7/mHPRT (Osterix derived from mouse), d: Results showing the relative expression of Alkaline phosphatase (mAlpl/mHPRT). (*) mSp7 and Alp are marker genes for indicating the osteoblast differentiation. Note that no significant change is observed for both the mSp7 and the Alp results.
Ⅳ. DISCUSSION
The effect of storage time tabulated in Table 1 shows that the carbon contamination increased gradually but significantly with the age of the microimplants. This result correlated with the result of Att W et al. (2009), and suggested that the carbon atoms (or molecules) in the air are absorbed by the oxide layers of the titanium microimplants in a continuous manner. It is noteworthy, that the newly produced microimplants showed a fair amount of carbon contamination already (50.86%). Given that the annual increase of carbon percentage across the eleven years is approximately 1.5%, this result indicated that most of the carbon contamination takes place during the production processes of the microimplants.
Autoclaving did marginally increase carbon content on the microimplant surfaces. As shown in Figure 1, a statistically significant increase in carbon percentage has occurred in the newly manufactured microimplants. This means that autoclaving plays a role in terms of carbon percentage and these results are in agreement with (Lausmaa J et al. 1990, Serro AP et al. 2003, Park JH et al. 2012). This result shows that even though in steam autoclaving the water is deionised, it can cause an increase in carbon contamination and deteriorate the biological properties of the microimplant surfaced. Vezeau et al. (1996), described this phenomenon is associated with the increase in the thickness of the oxide layer. As all microimplants need to be autoclaved before use, these results cannot be overlooked. On the other hand, the increase of carbon after autocalving of old microimplants was statistically insignificant. As a known fact one of the indicators of treated titanium surface is the reduction of the hydrocarbon. UV treatment removes carbon through two possible mechanisms; one by inducing photocatalytic activity of the TiO2 and another by the direct decomposition of carbon by UV per se (Aita H et al. 2009, Takeuchi M et al. 2005 & 2007). Figure 1 presents a clear explanation of the effects of UV treatment on the carbon percentage among newly manufactured and eleven years old microimplants. Note, the results of the current study show an overall reduction in the carbon percentage, which was statistically significant among both the newly manufactured and eleven year old microimplants. These results coincide with the study of Att W et al. (2009 & 2012) and Aita H et al. (2009) who showed that UV treatment reduced the hydrocarbon contamination over titanium surfaces.
Another known indicator of UV treatment is change in the surface charge of the titanium surface of microimplants. Microimplant surfaces are known to have a principal TiO2 layer. It is evident in Figure 2 that the autoclaved microimplant surface showed hydrophobicity as a result of which the water droplet retained on the surface with a contact angle of 74°. On the other hand, on the UV treated microimplant the water droplet spread over the surface with a contact angle of 10° indicating a change in the surface charge and exhibiting superhydrophilicity (Table 3). This result showed a statisitcally significant difference in the contact angle as an effect of the UV treatment over the microimplant surface. These result coincides with the results of Hirota et al (2014). UV treatment is known to alter the electrostatic properties of titanium surface to a positive charge (Hori N et al., 2010 and Jung-Hwan Lee et al., 2016), which could be benefitial for bone tissue formation and ossseconductivity on the titanium surface.
The results obtained from the cell culture (Figure 3a and 3b) showed that there was no difference visually in cell differentiation in the samples irrespective of whether they were treated with UV treatment or not. The gene expression results (Figure 3cc and 3d) confirmed this result. Hirota et al (2014), stated that “the change in the titanium surface properties by treatment could be used to obtain benefits for bone tissue formation and osteoconductivity on the titanium surface.” The current study contradicts their results, as the UV treatment did not show any major difference in the activities of osteoblasts over the UV treated titanium samples. The reason for this could be because of the difference in the surface texture. The titanium samples used in the present test lacked the extensive manufacturing process and had a smooth surface in contrast to the titanium mesh used by Hirota et al (2014) which received complex surface treatment similar to dental implants (Coelho PG et al. 2009, Novaes Jr AB et al. 2010).
In overall, among other new technology developed for dental implants Kim et al (2015), UV treatment can be used to decrease the carbon percentage of the microimplants irrespective of the age, by decontaminating the surface and reduce the disadvantages of the aging process and allowing a better bioactivity of the titanium surface, hence supporting the study of Hirota et al (2014) and Att W et al (2012). The reduction in carbon and the increase in hydrophilicity after UV treatment could lead to the promotion of osseointegration of the orthodontic microimplant. Yet Future studies to check the effectiveness of UV treatment on the bone to microimplant interface is recommended in-vivo.
Ⅴ. CONCLUSION
• The longer the storage time the higher was the carbon contamination over the microimplant surface.
• Steam autoclaving increased the carbon content on the microimplant surfaces which can decrease the hydrophilicity.
• UV treatment reduced the carbon content and enhanced the superhydrophilicity on the microimplant surfaces, although it did not result in substantial difference in osteoblastic differentiation.
References
-
Aita, H, Hori, N, Takeuchi, M, (2009), The effect of ultraviolet functionalization of titanium on integration with bone, Biomaterials, 30(6), p1015-25.
[https://doi.org/10.1016/j.biomaterials.2008.11.004]
-
Att, W, Hori, N, Takeuchi, M, (2009), Time-dependent degradation of titanium osteoconductivity: an implication of biological aging of implant materials, Biomaterials, 30(29), p5352-63.
[https://doi.org/10.1016/j.biomaterials.2009.06.040]
- Att, W, Ogawa, T, (2012), Biological aging of implant surfaces and their restoration with ultraviolet light treatment: a novel understanding of osseointegration, Int J Oral and Maxillofacial Implants, 27(November), p753-61.
-
Buser, D, Broggini, N, Wieland, M, (2004), Enhanced bone apposition to a chemically modified SLA titanium surface, J Dent Res, 83(7), p529-33.
[https://doi.org/10.1177/154405910408300704]
-
Byoung-Kook Kim, Kwang-Mahn Kim, Young Jin Kim, (2015), Patent analysis-based technology level evaluation of dental implant, Korean J Dent Mat, 42(1), p35-43.
[https://doi.org/10.14815/kjdm.2015.42.1.35]
-
Chin, MYH, Sandham, A, de Vries, J, van der Mei, HC, Busscher, HJ, (2007), Biofilm formation on surface characterized micro-implants for skeletal anchorage in orthodontics, Biomaterials, 28(11), p2032-40.
[https://doi.org/10.1016/j.biomaterials.2006.12.014]
-
Coelho, PG, Granjeiro, JM, Romanos, GE, Suzuki, M, Silva, NR, Cardaropoli, G, Thompson, VP, Lemons, JE, (2009), Basic research methods and current trends of dental implant surfaces, Journal of Biomedical Materials Research Part B: Applied Biomaterials, 88(2), p579-96.
[https://doi.org/10.1002/jbm.b.31264]
-
Haibin, L, Lei, W, Xueyang, Z, Mingdeng, R, Zehong, G, Lei, Z, (2013), Effects of Hydrocarbons Contamination on Initial Responses of Osteoblast-like Cells on Acid-Etched Titanium Surface, Rare Metal Mat Eng, 42(8), p1558-62.
[https://doi.org/10.1016/s1875-5372(13)60089-2]
- Hirota, M, Ikeda, T, Tabuchi, M, (2014), Effect of Ultraviolet-Mediated Treatment for Bone Formation Around Medical Titanium Mesh, J Oral Maxillofacial Surg, 72(9), p1691-702.
- Hori, N, Ueno, T, Minamikawa, H, et al , (2010), Electrostatic control of protein adsorption on UV-photofunctionalization titanium, Acta Biomater, 6, p4175.
- Jung-Hwan Lee, Soo-Kyung Jun, Hae-Hyoung Lee, (2016), Chair-side surface treatment method for inducing hydrophilicity in titanium dental implant, J Korean Dent Assn, 54(12), p985-995.
- Kim, Y-G, Park, J-W, Lee, J-M, Suh, J-Y, Lee, J-K, Chang, B-S, Um, H-S, Kim, J-Y, Lee, Y, (2012), IL-17 inhibits osteoblast differentiation and bone regeneration in rat, Archives of Oral Biology.
-
Lausmaa, J, Kasemo, B, Mattsson, H, (1990), Surface spectroscopic characterization of titanium implant materials, Applied Surface Science, 44(2), p133-46.
[https://doi.org/10.1016/0169-4332(90)90100-e]
-
Mouhyi, J, Sennerby, L, Pireaux, J-j, Dourov N, Nammour, S, and Van Reck, J, (1998), An XPS and SEM evaluation of six chemical and physical techniques for cleaning of contaminated titanium implants, Clin Oral Imp Res, 9, p185-194.
[https://doi.org/10.1034/j.1600-0501.1998.090306.x]
- Novaes, Jr A B, Souza, S L S, Barros, R R M, Pereira, K K Y, Iezzi, G, Piattelli, A, (2010), Influence of implant surfaces on osseointegration, Brazilian Dental Journal, 21(6), p471-481.
- Olefjord, I, & Hansson, S, (1993), Surface analysis of four dental implant systems, Int J Oral Maxillofacial Implants, 8(1).
-
Park, JH, Olivares-Navarrete, R, Baier, RE, (2012), Effect of cleaning and sterilization on titanium implant surface properties and cellular response, Acta Biomaterialia, 8(5), p1966-75.
[https://doi.org/10.1016/j.actbio.2011.11.026]
- Sul, YT, Johansson, CB, Petronis, S, (2002), Characteristics of the surface oxides on turned and electrochemically oxidized pure titanium implants up to dielectric breakdown: the oxide thickness, micropore configurations, surface roughness, crystal structure and chemical composition, Biomaterials, 23(2), p491-501.
- Serro a, P, Saramago, B, (2003), Influence of sterilization on the mineralization of titanium implants induced by incubation in various biological model fluids, Biomaterials, 24, p4749-60.
- Tabuchi, M, Ikeda, T, Nakagawa, K, (2015), Ultraviolet treatment increases removal torque values and horizontal stability of orthodontic miniscrews, Am J Orthod Dentofacial Orthop, 148(2), p274-82.
-
Takeuchi, M, Sakamoto, K, Martra, G, Coluccia, S, Anpo, M, (2005), Mechanism of photoinduced superhydrophilicity on the TiO2 photocatalyst surface, J Physical Chemistry B, 109(32), p15422-15428.
[https://doi.org/10.1021/jp058075i]
-
Takeuchi, M, Martra, G, Coluccia, S, Anpo, M, (2007), Verification of the photoadsorption of H2O molecules on TiO2 semiconductor surfaces by vibrational absorption spectroscopy, J Physical Chemistry C, 111(27), p9811-9817.
[https://doi.org/10.1021/jp0689159]
-
Vezeau, PJ, Koorbusch, GF, Draughn, R a., Keller, JC, (1996), Effects of multiple sterilization on surface characteristics and in vitro biologic responses to titanium, J Oral Maxillofacial Surg, 54(6), p738-46.
[https://doi.org/10.1016/s0278-2391(96)90694-1]